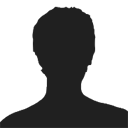
伤口世界
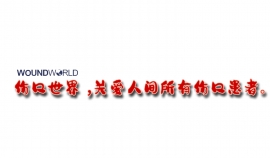
- 星期五, 23 9月 2022
Cellular Derivatives and Efficacy in Wound and Scar Management
Albertine Lapp1 , Pascal Furrer1 , Albert-Adrien Ramelet2 , Christian Aubort3 , Pierre Aubort3 ,Philippe Laurent1,4, Lee Ann Applegate4,5
1Department of Pharmaceutical Sciences, University of Geneva, Geneva, Switzerland;
2 Office of Dermatology and Angiology,Lausanne, Switzerland;
3 Sincopharm, Moudon, Switzerland;
4 Tec-Pharma, Bercher, Switzerland;
5 Department of Plastic and Reconstructive Surgery, University Hospital, Lausanne, Switzerland.
Email: 该Email地址已收到反垃圾邮件插件保护。要显示它您需要在浏览器中启用JavaScript。
Received November 15th, 2012; revised December 18th, 2012; accepted December 27th, 2012
ABSTRACT
Biologicals have been used for decades in biopharmaceutical topical preparations. Because cellular therapies are routinely used in the clinic they have gained significant attention. Different derivatives are possible from different cell and tissue sources, making the selection of cell types and establishment of consistent cell banks crucial steps in the initial whole-cell bioprocessing. Various cell and tissue types have been used in treatment of skin wounds including autologous and allogenic skin cells, platelets, placenta and amniotic extracts from either human or animal sources. Experience with progenitor cells show that they may provide an interesting cell choice due to facility of out-scaling and known properties for wound healing without scar. Using defined animal cell lines to develop cell-free derivatives may provide initial starting material for pharmaceutical formulations that help in overall stability. Cell lines derived from ovine tis sue (skin, muscle, connective tissue) can be developed in short periods of time and consistency of these cell lines was monitored by cellular life-span, protein concentrations, stability and activity. Each cell line had long culture periods up to 37 - 41 passages and protein measures for each cell line at passages 2 - 15 had only 1.4-fold maximal difference. Growth stimulation activity towards two target skin cell lines (GM01717 and CRL-1221; 40 year old human males) at concentrations ranging up to 6 μg/ml showed 2-3-fold (single extracts) and 3-7-fold (co-cultured extracts) increase. Proteins from co-culture remained stable up to 1 year in pharmaceutical preparations shown by separation on SDSPAGE gels. Pharmaceutical cell-free preparations were used for veterinary and human wounds and burns. Cell lines and cell-free extracts can show remarkable consistency and stability for preparation of biopharmaceutical creams, moreover when cells are co-cultured, and have positive effects for tissue repair.
Keywords: Biological; Wound Healing; Scars; Cell-Free Derivatives
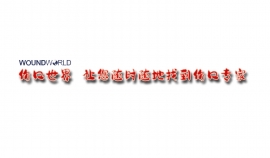
- 星期二, 20 9月 2022
Conductive Biomaterials as Bioactive Wound Dressing for Wound Healing and Skin Tissue Engineering(3)
5.1.2 3D Conductive Biomaterials for Acute Wound
3D biomaterials including hydrogels, foams, and sponges possess great potential in assembling ECM-like structure, so they have attracted much more attention in wound dressing and skin tissue scaffolds. Since there are diverse fabrication methods that can circumvent the limitations of these conductive substances, all types of conductive substances have been incorporated into various forms of 3D biomaterials and proved their merits in acute full-thickness wound treatment.
Compared with 2D biomaterials, 3D biomaterials owning highly interconnected porous structure demonstrate several advantages. Bioactive agents including drugs and growth factors can be easily loaded into 3D biomaterials and exhibit sustained release profiles, which can benefit would healing [183, 185]. The higher water absorption capacity makes 3D biomaterials fit for wounds with large exudate and avoid
frequent removal. The injectability and self-healing capacity at ambient environment make the hydrogel-based wound dressing suitable for irregular and deep wounds [171, 174,181, 189]. Moreover, the mechanical properties of hydrogels could be easily adjusted to have suitable modulus and highly stretchable to comply with wounds at any part of the body, especially for wounds under large and incessant movement [171, 174, 179, 195]. Recently, Li et al. presented a conductive hydrogel based on PEDOT:PSS and guar slime, and verified its application on wounds in stretchable parts of the body [186]. The hydrogel exhibited rapid gelation
within 1 min, injectability and self- healing ability. Compared with the dorsum of rats mostly being in static, nape is in frequent movement including compression, tension and twist. As shown in Fig. 12a, b, the designed dressings were applied on wounds constructed on the nape and dorsum of rats. Obviously, large movement would lead to delayed healing process. But when treated with a compliant conductive hydrogel-based wound dressing, wounds on the nape demonstrated an improved healing process according to the statistical data summarized in Fig. 12c e. For this reason, 3D conductive biomaterials with compliance and high adhesiveness have paved way for the treating of wounds under incessant movement, for they could not adhere tightly to the wound without extra assistant, but also maintain structural integrity supporting full coverage for wound bed.
Due to the low adhesiveness, traditional wound dressings and novel 2D biomaterial-based wound dressings always require additional medical tape to be retained in wound sites. Large wounds often need commercial adhesives to promote wound closure and healing. Some novel adhesive conductive hydrogel-based wound dressings solve these two issues and improve the hemostatic effect at the same time. Conductive hydrogels containing Schiff base [174, 181] or polydopamine [179, 180] have been proven with high tissue adhesiveness, comparable or even better to that of commercial dressings. To combine the advantages of conductive biomaterials and adhesiveness, our group developed an injectable, self-healing hydrogel (QCS/rGO PDA/PNIPAm), containing PDA and QCS for antibacterial properties and strong adhesiveness, PNIPAm for biomechanical activities, and rGO for electroconductivity [185]. Eventually, this hydrogel significantly promoted the full-thickness wound healing process demonstrating higher granulation tissue thickness, collagen disposition, and enhanced vascularization. The enhanced wound healing effect of this conductive hydrogel could be ascribed to accelerated wound closure through biomechanical adhesiveness and multiple biochemical functions simultaneously.
Conductive hydrogels can also work as electrode to promote the efficiency of electrotherapy in curing full-thickness wounds. Mao et al. employed a regenerated bacterial cellulose/MXene composite hydrogel as the wound dressing and electrode for ES [141]. The composite hydrogel with 2% MXene content demonstrated the highest electrical conductivity, the best biocompatibility, and suitable mechanical
properties. By in vitro electrostimulated cell culture assay and in vivo animal assay, this conductive hydrogel containing MXene was found to remarkably promote wound healing by applying 100 mV mm-1 DC electric field strength for 1h every two days via wound contraction analysis and histopathologic evaluations, as illustrated in Fig. 13. Overall, due to the versatile structures with high tolerance to accommodate multiple functions and properties, 3D conductive biomaterials have made great achievement in wound healing, especially for acute wounds.
5.2 Chronic Wound
Chronic wound, including arterial, diabetic, pressure, and venous ulcers, is a serious threat to human health, and it takes decades to heal and accompanies by severe complication, amputations and even death [10, 33]. Traditional passive wound dressings are not effective enough for wound care of chronic wounds, because they could only provide protection against from exposure and moist balance [35, 67,145]. The tissue debridement and infection control need further surgery and drug delivery. Overall, novel 3D conductive biomaterials integrating wound care and treatment have been paid much attention and need further development. Infected wound is one classic type of chronic wounds. Ideally, asepsis wounds will pass through the inflammatory phase after 2- -5 days and gradually proceed into the proliferation and remodeling phases. Excessive and prolonged inflammation is obnoxious inevitably results in delayed healing and even death [231]. Actually, chronic wounds including diabetic wounds and ulcers could hardly proceed beyond the inflammatory phase [232]. Disinfection of infected wounds and prevention of wounds from bacteria invasion during the entire healing procedure are both essential for wound management [233]. Conductive biomaterials certainly exert positive effects during the inflammatory phase ascribing to their inherent antibacterial activities and photothermal antibacterial properties if necessary, thus prompting the transition to the proliferation phase. Besides, conductive biomaterials have been proved to exhibit antioxidant activity and enhance cell attachment, migration, and proliferation, which benefits both the inflammatory, proliferation, and remodeling phases [234]. In addition, when applied as electrodes in electrical therapy, conductive wound dressing can improve cell migration, alignment, proliferation and differentiation with specific programmed electrical stimulation [235]. In overall,conductive biomaterials could enhance wound healing through multiple pathways. Nevertheless, considering the complex in different wounds especially for chronic wounds, conductive biomaterials need to be tailored with multifunction or combined with other specific bioactive agents.
5.2.1 Infected Wound
Bacterial infection has long been a severe threat to human health. On one hand, they could induce many diseases and increase more complication during the treatment. On the other hand, bacterial resistance caused by abuse of antibiotics continues presenting significant burden on public health [54, 236, 237]. Wound infection is one of these tough issues [238]. Microorganisms can invade wounds and induce inflammation. Rapid colonization and the biofilms would prevent re-epithelization, and prolong wound healing process, and eventually lead to chronic bacterial-infected wounds [10]. Besides, another issue antibiotics suffering is that they could hardly penetrate biofilms, thus resulting in poor antibacterial efficiency [239]. Fortunately, conductive substances including CPs [201, 240], carbon nanomaterials [177, 180, 183], metals and metal oxides [123], MXene [143], and BP [177] exhibiting intrinsic antibacterial and photothermal antibacterial activities are all good alternatives for antibiotics, because they are less prone to induce bacterial resistance. Commonly, they can be solely incorporated into nonconductive polymeric matrix, exerting excellent bacterial killing effect and promotion toward infected wounds [143, 177, 201, 241]. Among various matrix materials, chitosan and its derivatives have been frequently selected for their synergistic intrinsic antibacterial effect. Chitosan derivatives as N-carboxyethyl chitosan and quaternized chitosan have also been combined with GO [187] or CNT[183] in designing conductive hydrogel-based wound dressings. These conductive dressings demonstrated multifunctional features and realized higher degrees of wound closure and skin regeneration within 14 days.
Conductive materials with nanostructure morphology owning increased membrane permeability and multiple antibacterial actions, are other preferential choices to deal with infected wounds [242]. In addition to the above -mentioned carbon-based nanomaterials that have been widely applied in infected wound management, nanometer-scaled conductive materials including CPs, metals and metal oxides, and semiconductors also show great value in promoted antibacterial efficiency. However, free nanomaterials are likely to be cleared rapidly from the interstices of tissues once being implanted owing to their small size [243]. Sung group reported chitosan derivatives containing self-doped polyaniline could self-assemble into nanostructures [240]. As shown in Fig.14, polyaniline-conjugated glycol chitosan (PAN-GCS) could spontaneously form nanoparticles in aqueous solution. Since the surface charge of PANI-GCS NPs was sensitive to surrounding environment, these PANI-GCS NPs suffered a bacterium-specific aggregation induced
by localized skin infections which possessing acidic pus, while exerting no influence toward healthy tissues. By this method, the retention capability of PANI-GCS NPs at the injection area was significantly improved. Moreover, under NIR irradiation, there exhibited specific heating of PANI- GCS NPs/bacteria aggregates, the temperature dramatically reached 55 ℃, whereas a slight increase to 33 C of the surrounding normal tissue. Presently, the encapsulation of conductive nanomaterials into multifunctional platform and combination with other bioactive agents have become necessary to implement their application in vivo.
Compared with antibacterial agents including Zn2+ and Cu2+ with narrow antibacterial spectrum, short-term durability, low heat resistance and stability, metal oxides as ZnO and CuO in nanostructure exhibit improved antibacterial capability, thereby possess great potential in curing infected wounds. Besides, due to the excellent antibacterial ability and extraordinary photothermal effect, Au NPs have excellent performance in killing bacteria. Wang et al. developed a polyvinyl alcohol composite film embedded with hybrid multi-shelled nanoparticles (ZnO@CuO@ Au NPs) [123]. Under NIR laser irradiation, this composite film demonstrated enhanced ROS generation, destruction of bacterial cell membranes, and antibacterial efficacy, ascribing to the photothermal and photodynamic effect, and sustainably released Zn2+/Cu2+. Excitingly, MXene nanosheet as a novel class of 2D inorganic compounds of metal carbides and carbonatites with excellent conductivity, biocompatibility, and antibacterial ability, has shed light on the treatment of infected wounds, as reported by Zhang group [143]. Also, a BPs nanosheets- incorporated chitosan hydrogel has proved its effectiveness in treating S. aureus-infected skin wounds due to the production of singlet oxygen under simulated visible light, compared with pure chitosan hydrogel [177].
Combination of conductive materials and antibiotics is also a commonly used strategy for managing infected wounds. The synergistic effect from different antibacterial materials can not only reduce the drug resistance and ensure the antibacterial performance, but also alleviate the abuse of antibiotics. The drug -resistant methicillin resistant staphylococcus aureus (MRSA)-infected wound model is well established to evaluate the efficiency of conductive biomaterials. Antibiotics, as doxycycline [180, 187] and moxifloxacin hydrochloride [183] with resistance toward MRSA have been combined with GO or CNT. The hydrogel
matrices allowed for controlled and sustained release profile of antibiotics. Moreover, due to the efficient penetration of nanomaterials through biofilm, the antibiotic-loaded hybrid nanomaterials would largely increase the local concentration of antibiotics in the biofilm. Altinbasak et al. presented a rGO embedded PAA nanofiber mat, and antibiotics were simply loaded through immersion. This composite mat exhibited low passive diffusion-based release at ambient environment, whereas realized “on-demand" release tuned by power density of applied irradiation. Indeed, these hybrid nanofiber mats with photothermal assistance demonstrated the supreme wound healing capability of MRSA- infected wounds. Despite the promising achievement in infected wounds, the fact should not be overlooked that conductive biomaterials are usually synergistically combined with antibiotics and photothermal therapy. Moreover, whether conductive biomaterials are effective enough to severely infected wounds with biofilms still needs further investigation.
5.2.2 Diabetic Wound
Compared with acute wounds, diabetic wounds exhibited prolonged infection, abnormal angiogenesis, and delayed reepithelization. Therefore, the general principle of designing wound dressings and scaffolds for diabetic wounds is to prevent bacterial infection, control wound infection, induce angiogenesis, enhance collagen deposition, and promote cell proliferation [10, 74]. Even though conductive biomaterials have achieved excellent treatment effects on acute wounds and infected wounds, they are rarely used alone when dealing with diabetic wounds [1 82, 219]. Notably, conductive biomaterials encapsulated with insulin and fibroblast [244], or with mesenchymal stem cells have demonstrated enhanced diabetic wound healing performance [206, 207, 245]. Jin et al. presented an injectable conductive hydrogel based on aniline tetramer which could promote diabetic wound healing by incorporation of laccase to cast a hypoxic microenvironment maintaining for 13 h [184]. Such a hypoxia- pretreatment would largely enhance the effectiveness of adipose- derived mesenchymal stem cells when treating diabetic wounds. Subsequent adequate oxygen supply is essential for diabetic wound healing; thus, it is highly necessary to delivery oxygen to wound sites in a sustained and controlled manner. Zhang et al. developed BP contained thermo-responsive microneedles composing of polyvinyl acetate film as the backing layer and gelatin hydrogel loaded with BP quantum dots and hemoglobin as the tip, as shown in Fig. 15 [191]. Combining the photo thermal effect of BP quantum dots and reversible oxygen binding property of hemoglobin, these microneedles realized NIR-controlled oxygen delivery. Under programmed intermitted NIR irradiation, these microneedles could support adequate oxygen supply lasted for 24 h. Indeed, these multifunctional microneedles demonstrated enhanced wound healing when treating full-thickness diabetic wound. On day 9, the group treated with BP quantum dots and NIR irradiation demonstrated most advanced healing performance, in terms of wound closure, granulation tissue width, epithelial thickness, and blood vessel density.
Electrotherapy has positive effects to treat chronic wounds but is still limited by small area of electrodes and uneven distribution of ES. Lu et al. have proved that applying conductive biomaterials as the electrodes in ES strategies could drastically improve the efficiency of electrotherapy [50]. Zhang et al. created a conductive self-healing hydrogel based on Zn2+ and PPy [190]. The group of diabetic wounds covered with the conductive hydrogel and stimulated by a direct current voltage of3 V for 1 h per day demonstrated the optimum wound healing performance than the group only covered with hydrogel and the control group. Overall, conductive biomaterials have demonstrated excellent performance in managing diabetic wounds though different pathways. Still, considering the variety and complexity of
chronic wounds, the usage of conductive biomaterials, their combination with other reagents and specific implementation approaches need to be further explored [246].
5.3 Wound Monitoring
Wound healing is a dynamic process comprising four overlapped stages, in which many parameters including humidity, temperature, pH, and glucose levels will change [247]. Compared with healthy skin, wounds demonstrate typical differences according to their types. On one hand, these differences could be utilized to design smart wound dressings that can specifically react to wounds but are inert to healthy skin [232, 239]. So far, numerous wound dressings with stimuli-responsiveness have been developed which can actively sense the variations and then self-adapt to the wounds. However, stimuli-responsive conductive wound dressings have not been widely explored [248]. At present,stimuli-responsive conductive wound dressings can be classified into two categories according to the sources of the two features. The first method is employing two distinct functional groups that endow wound dressings with stimuliresponsiveness and conductivity, correspondingly. Thus, the evaluation of stimuli-responsiveness and conductivity and their effects on wound healing could be studied separately. Zhao et al. reported a multifunctional hydrogel dressing consisting of boronate-based dynamic network and conductive component Ag NWs [192]. The boronate-based dynamic network would collapse when treated with glucose, which benefits diabetic foot wound healing by facile on-demand removal. Eventually, wounds treated with this hydrogel dressing demonstrated rapid wound contraction rate and lower glucose level. Our group developed a hydrogel wound dressing exhibiting pH -responsiveness derived from Schiff base based network and conductivity from CNT [183]. The pH-responsiveness is conducive for controlled drug release when treating infected wounds which exhibiting acidic pH. In the second method, both stimuli responsiveness and conductivity are derived from the same substance. Sung et al. synthesized a conductive chitosan derivate grafted with mercaptopropylsulfonic acid-doped polyaniline (NMPA-CS) and applied this derivate in treating infected wounds [201]. The CS derivative would self- assemble into micelles in acidic aqueous and form colloidal gel when increasing pH to 7.0. Thus, when injected into an infected wound, the NMPA-CS solution will completely cover the acidic area until gelation occurs when encountering healthy tissue. In their subsequent work, PANI-GCS NPs demonstrating positive charge under acidic environment can form aggregation with negatively charged bacteria, further facilitating photothermal ablation of focal infection [240]. Since the conductivity of CPs would be significantly affected by pH, the conductivity of the dressings would also change When the wound dressings undergo a specific change after sensing this stimulus [249].
On the other hand, wound monitoring has been developed. Diagnosis and monitoring of wounds are very imperative, especially for chronic wounds [6, 228]. Physical examination and the parameters including wound location, size, depth, and drainage should be well recorded and further treatment needs to be adjusted depending on the healing degree. However, long-term monitoring relies on patients' hospitalization and frequent screening. Moreover, visual evaluation is far from accuracy and promptness. Some electrochemical sensors have been designed for wound diagnosis, but the wound dressing and wound treatment could not complete simultaneously [232, 250, 251]. Conductive wound dressings which can sense the wound variations and then convert them into electrical signals enabling synchronous wound care and wound monitoring are of great value in modern wound care. Recently, Zhao et al. fabricated an antibacterial conductive hydrogel as wound dressing based on polydopamine, AgNPs, PANI, and PVA [182]. This hydrogel could directly adhere to human skin and respond to human mechanical deformation. Excitingly, they found that this hydrogel could distinguish diabetic rats from normal rats by movement, because diabetic rats have a relative slower respond to thermal stimuli. Jia et al. fabricated a PEDOT coated conductive silk microfiber integrated patch, and then employed this patch as ECG and EMG electrode for diagnosis in diabetes while promoting wound healing [168].
Another characteristic of chronic wound is the pH value. Compared with healthy skin with acidic pH ranging5.5- 6.5, chronic wounds exhibit alkaline pH between 7 and 9 or extremely acidic pH by severe infection [252, 253]. The level of pH can be continuously measured to monitor the chronic wound healing process. Recently, several works reported conductive biomaterials that can be used both as wound
dressing and sensor. PANI is a proton-selective polymer and the conductivity of PANI is depended on protonation and deprotonation under different conditions [77, 82, 132]. Thus,PANI can be used to fabricate pH sensors monitoring wound status [254]. Mostafalu et al. developed a wound dressing integrating PANI-based pH sensors and flexible microheater with alginate hydrogel loaded with thermo-responsive drug
carriers for antibacterial drug [255]. The dressing was also assembled with a wireless Bluetooth module for real time monitoring, as illustrated in Fig. 16.
Glucose level is also a key factor of the diabetics. Lipani et al. reported a graphene-based thin film integrated with an electrochemical glucose sensor and proved this assembly platform could be applied as a noninvasive, transdermal glucose monitoring system to track blood sugar in human [250]. Thus, it could be anticipated with the emergence of wound dressing integrated real-time glucose sensing system in the future.
So far, real-time tracking of wound healing has been realized through monitoring the level of several parameters, including physiological signals [168], pH [193, 249, 251,252, 254], oxygen [19], temperature [239], moisture [256], glucose [193, 250], and uric acid [257]. We envision that more comprehensive portable healthcare devices with high accuracy and precision based on conductive biomaterials will be manufactured, guaranteeing suitable wound care and noninvasive real-time healing measurement with compre-hensive adaptivity at the same time.
6 Summary and Perspectives
This review summarizes the application and achievement of conductive biomaterials in wound healing and skin tissue engineering. Conductive substances including CPs and their oligomers, carbon nanomaterials, metals and metal oxides, and novel 2D inorganic nanomaterials all have great advantages and serious drawbacks. CPs are limited by the poor processability, mechanical brittleness and nonbiodegradability, and the conductive oligomers benefit manufacture process and good biodegradability, but their conductivity under physiological conditions restricts further practical applications. Carbon nanomaterials and metals and metal oxides tend to aggregate in solution, and the homogeneous dispersion always requires aid from other polymers or techniques. The cytotoxicity of carbon nanomaterials and metals and metals oxides also matters their applications, especially in some researches that they are modified with CPs. More importantly, the conductivity of these materials dependeds on many factors, including pH value, dopant, and adjacent environment. But, in most articles, the measurement for the conductivity of biomaterials was taken place at specific conditions, which are totally different from the real conditions. BPs and MXene with great opportunities in wound healing are still facing a myriad of challenges before fulfilling application in wound healing.
Conductive biomaterials can be fabricated into diverse forms to meet the requirements of different types of wounds. 2D biomaterials as films, micro- and nanofibers, and membranes can treat acute wounds with fewer exudates, while 3D hydrogels and scaffolds with ECM-like structures are widely used in more complicated wounds and skin substitutes. Amidst, conductive thin films and hydrogel membranes can also work as substrates for bioelectronics, due to the soft feature, flexibility, and suitable mechanical properties [258- -260]. Conductive film and micro-/nanofibers commonly possess much limited water-uptake ability,
and the conductivity is always measured in the dry state. Therefore, they are suitable for wounds with low exudates. Conductive hydrogels and 3D porous scaffolds have high water-uptake ability, and the conductivity in dry and wet states both should be measured because of the ionic conductivity dominating in the wet state. Conductive hydrogels fit for wounds with moderate exudates, while 3D porous scaffolds can manage significant exudates. Moreover, conductive fibers, hydrogel, and 3D porous scaffolds can be loaded with drugs, growth factors, and cells, thus demonstrating great competitiveness in the multifunctional platform and even engineered skin substitutes.
Conductive biomaterials realize their applications in promoting wound healing via three strategies. First, they can be applied as compliant electrodes for electrotherapy. In general, the conductive wound dressings can facilitate ES to be well and uniformly conducted onto the wounds promoting the efficacy of electrotherapy [50, 109, 120, 225,226]. Second, they can be used alone as wound dressings
or tissue engineering scaffolds, demonstrating similar conductivity to human skin and supporting cellular activities, to accelerate wound healing performance. In addition, some conductive wound dressings loaded with bioactive agents have achieved controlled drug release assisted by an external circuit, realizing long-term treatment and lowering the initial burst and side effects [130, 131, 261- 266]. In the absence of
external ES, these conductive biomaterials still demonstrate enhanced cell attachment and proliferation, and promoted wound healing performance [37]. Also, the effectiveness of conductive biomaterials in promoting wound healing can be attributed to the inherent antibacterial and antioxidant capacities and photothermal properties of these conductive substances [77, 139, 149, 267]. Third, conductive biomaterials could be manufactured into stretchable and flexible electronics for real-time monitoring of wound status. With the significant progress and achievement of flexible electronics and wearable smart biomedical devices, scientists have managed to integrate conductive wound dressing with wound diagnosis and monitoring capacity [249, 255]. Such advance has extremely facilitated wound healing, because it can avoid frequent removal and replacement for closer observation.
In contrast to visual and subjective observation, the instant automated evaluation with objective standards throws light on a more systematic analysis of wound healing and communication between patients and doctors. In many cases, bioactive agents, including drugs, proteins, and growth factors have been incorporated into conductive biomaterials to enhance wound healing performance through versatile methods. The synergistic effects vary and should be discussed in the specific situation by considering the inherent properties of these bioactive agents, the interactions between bioactive agents and conductive materials or the polymeric matrixes, and the external conditions. Fibronectin as cell- adhesive glycoprotein was incorporated into PPy/PLLA film to endow the conductive film with enhanced fibroblasts adhesion and migration, while incorporation of BSA leading to reduced cell adhesion [106]. The analgesic and anti-inflammatory drug ibuprofen has been loaded into a PPy-based conductive film through electro-chemical polymerization and realized on-demand, electronically controlled release under an electrical potential [130]. Curcumin, an effective drug demonstrating antioxidant, anti-inflammatory and antimicrobial activities, has long been limited by its hydrophobic nature [268]. Through the π - π interaction with aromatic ring in PANI, curcumin was entrapped within PHBV-g PANI composite film [114]. This curcumin entrapped conductive film does not only reveal small burst release and controlled drug release profile due to the noncovalent interaction, but also demonstrate enhanced differentiation, proliferation, and migration of fibroblast cells ascribing to the incorporation of curcumin. Stem cells exhibited self- renewal ability and could differentiate into multiple types of cells, and their availability in promoting wound healing and skin tissue engineering has been widely validated [269]. Bone marrow derived mesenchymal stem cells have been loaded with a graphene foam [205]. The conductive scaffold was biocompatible and conducive for growth and proliferation of bone marrow derived mesenchymal stem cells. Eventually, the cell-loaded conductive foam was found upregulating vascular endothelial growth factor and basic fibroblast growth factor resulting in reduced scar formation in full- thickness defect experiment. The wound-specific delivery of growth factor is of great value in promoting wound healing efficiency, because the low concentration and excessive degradation of growth factor on wound site would delay the healing process [270]. Metal ions are of great value in maintaining human activities, wound healing process included. Copper ions and zinc ions have been incorporated into PEDOT-cellulose polymer composite through doping mechanism without affecting roughness topography of the substrate and realized controlled release. The combination of PEDOT with metal ions on cellulose substrate eventually contributed to enhanced attachment and proliferation of human keratinocytes [119]. Growth factors modulate a series of cellular activities for many types of cells, which is crucial in wound healing [64, 271]. Human keratinocytes' directional migration under electric fields requires several growth factors, particularly epidermal growth factor [39]. In contrast to plenty of works about growth factors loaded conductive biomaterials for cardiac, muscle, and nerve tissue engineering [14, 270, 272, 273], the application of growth factors loaded conductive biomaterials in wound healing and skin tissue engineering has not been widely reported yet [274, 275]. Epidermal growth factor was loaded into a conductive polyacrylamide/chitosan hydrogel. Due to the coexistence of PPy nanorods and epidermal growth factor, the composite hydrogel demonstrated the optimal wound healing effects [176] Based on the above facts, the combination of growth factors and conductive biomaterials is highly appreciated. The ways by which growth factors and conductive biomaterials combined, the interactions between them, and their application in specific types of wound healing need to be further explored.
In addition, the applications of conductive biomaterials are still in the preliminary stage, limited in acute wound, infected wound and diabetic wound. Whether conductive biomaterials wound promote the healing process for other types of wounds needs to be explored in the next, as well as the detailed mechanisms. Furthermore, there have not established some standard principles to compare the wound
healing effects of these conductive biomaterials, as there are various animal models and different types of wounds. Even if in some works, the selected control groups as traditional passive wound dressings seem not convincing enough, because the chosen control group should be in the same morphology as the designed conductive biomaterials. Another issue is about the manufacturing process. The synthesis and incorporation of conductive substance are often complicated and sometimes require harsh conditions, which would restrict their further application and induce environment issues. Conductive substances are always combined with other functional materials. The interactions including synergistic effect or related side effects also should be fully evaluated.
So far, there are several commercially available health care products that contains silver ions, such as ACTICOAT antimicrobial silver dressing, AQUACEL@ Ag foam, Biatain Silicone Ag, and SILVERCELTM nonadherent dressing. But, these commercial dressings are mainly claimed for the antimicrobial capability. PosiFect RD@ and Procellera@ are wearable bioelectric dressings and have received FDA approval. They can provide electrical stimulation to wound. However, there are no commercially available conductive biomaterials based on other conductive agents for wound healing and skin tissue engineering. Since the application of conductive biomaterials as wound dressings for wound healing and skin tissue engineering is in the very preliminary stage, there are still many challenges for the further application in practice and clinic. Biocompatibility is one of the important criteria for biomaterials. In vitro short-term blood compatibility and cytotoxicity to different fibroblasts and keratinocytes are the most frequently used methods to evaluate their biocompatibility. However, there lacks the study of long-term histocompatibility of these conductive biomaterials. The biodegradation mechanisms of these conductive biomaterials under physiological environment are not all clear yet. The stability of MXene nanosheets and BP nanosheet under physiological environment is questionable. The conductivity of these conductive biomaterials is always measured under an ideal stable condition that is totally distinct from the real physiological environment. The real conductivity of these conductive biomaterials under practical conditions, how the conductivity would change along with the degradation and upon hydration or dehydration, whether the conductivity would surpass the safe range are still under question. Beyond that, surface modification is indispensable for majority conductive materials. However, it would significantly alter the properties of conductive nanomaterials, including conductivity, hydrophilicity, surface morphology, and photothermal effect, which are all crucial in wound healing. Even worse, metal and metal oxides, BP, and MXene lack of functional surface groups, which make this issue challenging. Moreover, almost all in vivo experiments were conducted on murine defects. But there exists great difference between human and murine skin. Even though the current research results on murine are encouraging, more systematic study and exploration must be conducted for the detailed mechanism in terms of each wound healing phase, while employing other large animal models to further verify the potential application in clinic.
In summary, the general method to fabricate conductive biomaterials is to incorporate small amount of conductive substance within other nonconducting polymers, and the properties of conductive biomaterials are mainly depended on the selection of the matrix polymers and crosslinking methods. Meanwhile, to accelerate the wound healing process in multiple channels, combination conductive biomaterials with other bioactive agents and cells is an effective method and needs more exploration. Moreover, with the development of nanogenerators and bioelectronics, electrotherapy and real-time wound assessment assisted by conductive biomaterials will make significant progress in the next decades. Working as wound dressing or electrode, conductive biomaterials have made significant achievement in wound healing, skin tissue regeneration and real-time wound diagnosis. Based on these achievements and the booming development of new technology, we expect that conductive biomaterials would make more advanced development for wound healing.
Acknowledgements This work was jointly supported by the National Natural Science Foundation of China (Grant Numbers: 51973172, and 51673155), the Natural Science Foundation of Shaanxi Province (No.2020JC-03 and 2019TD-020), State Key Laboratory for Mechanical Behavior of Materials, and the Fun-damental Research Funds for the Central Universities, and the World-Class Universities (Disciplines) and the Characteristic Development Guidance Funds for the Central Universities, and Opening Project of Key Laboratory of Shaanxi Province for Craniofacial Precision Medicine Research, College of Stomatology,
Xi' an Jiaotong University (No. 2019LHM-KFKT008, and No. 2021LHM-KFKT005).
Funding Open access funding provided by Shanghai Jiao Tong University.
Open Access This article is licensed under a Creative Commons Attribution 4.0 International License, which permits use, sharing, adaptation, distribution and reproduction in any medium or format, as long as you give appropriate credit to the original author(s) and the source, provide a link to the Creative Commons licence, and indicate if changes were made. The images or other third party material in this article are included in the article's Creative Commons licence, unless indicated otherwise in a credit line to the material. If material is not included in the article's Creative Commons licence and your intended use is not permitted by statutory regulation or exceeds the permitted use, you will need to obtain permission directly from the copyright holder. To view a copy of this licence, visit http:/creativecommons. org/licenses/by/4.0/.
References
1. M. Colonna, Skin function for human CD1a-reactive T cells. Nat. Immunol. 11, 1079-1080 (2010). htps://oi.or/10.1038/i1210-1079
2. F. Strodtbeck, Physiology of wound healing. Newborn Infant Nurs. Rev. 1(1), 43- -52 (2001). htps://oiog/10.1053/obin. 2001.23176
3. D. Harper, A. Young, C.E. McNaught, The physiology of wound healing. Surg. Infect. (Larchmt.) 32(9), 445- 450 (2014). htps://doiog/10.1016/j.psur.2014.06.010
4. B.K. Sun, Z. Siprashvili, P.A. Khavari, Advances in skin grafting and treatment of cutaneous wounds. Science 346(6212), 941-945 (2014). htps:/oi.or/0.126/cien ce.1253836
5. S.K. Nethi, S. Das, C.R. Patra, S. Mukherjee, Recent advances in inorganic nanomaterials for wound-healing applications. Biomater. Sci. 7(7), 2652- 2674 (2019). hts://doi. org/10.1039/c9bm00423h
6. M.P Rowan, L.C Cancio, E.A. Elster, D.M. Burmeister, LF. Rose et al., Burn wound healing and treatment: review and advancements. Crit. Care 19, 243 (2015). htps://doior//./ 1186/s13054-015-0961-2
7. CK. Sen, Human wound and its burden: updated 2020 compendium of estimates. Adv. Wound Care 10, 281- 292 (2021). https://doi.og/10. 1089/wound.2021.0026
8. S.R. Nussbaum, M.J. Carter, C.E. Fife, J. DaVanzo, R Haughtm et al, An economic evaluation of the impact, cost, and medicare policy implications of chronic nonhealing wounds. Value Health 21(1), 27- -32(2018). https:/doi.org/ 10.1016/jjval.2017.07.007
9. J.G. Powers, L.M. Morton, TJ. Phillips, Dressings for chronic wounds. Dermatol. Ther. 26(3), 197- 206 (2013). htps://doi. org/10.111/dth. 12055
10. G. Han, R. Ceilley, Chronic wound healing: a review of current management and treatments. Adv. Ther. 34, 599- -610 (2017). htps://i.org/10. 1007/s12325-017-0478-y
11. s. Singh, A. Young. C.E. MeNaught, The physiology of wound healing Surg (Oxford) 35(9), 473- 477 (2017). http:/ doi.org/10.1016/j.mpsur.2017.06.004
12. S. Mandla, LD. Huyer, M. Radisic, Review: multimodal bioactive material approaches for wound healing. APL Bioeng. 2(2), 021503 (2018). htps://doi.org/10.1063/1 .5026773
13. G.S. Schultz, J.M Davidson, R.S. Kirsner, P Bornstein, L.M.Herman, Dynamic reciprocity in the wound microenvironment. Wound Repair Regen.19(2), 134-148 (201 1). https:/
doi.org/101111j.1524-475X.201 1.00673.x
14. J. Li, Y.P. Zhang, R.S. Kirsner, Angiogenesis in wound repair: angiogenic growth factors and the extracellular matrix. Microsc. Res. Tech. 60(1), 107-114 (2003). htps://oi.o/g// 10.1002/jemt. 10249
15. A.E. Rivera, J.M. Spencer, Clinical aspects of full-thickness wound healing. Clin. Dermatol. 25, 39 -48 (2007). ht://doi.org/10. 101 6/j.clindermatol.2006.10.001
16. M Madaghiele, C. Demitri, A. Sannino, L. Ambrosio, Polymeric hydrogels for burn wound care: advanced skin wound dressings and regenerative templates. Burns Trauma 2(4),153-161 (2014). htps://doi.org/10.4103/2321-3868.143616
17. L. Ambrosio, The role of biomaterials in burn treatment. Burns Trauma 2(4), 150-152 (2014). https://doi.org/10101/2321-3868.143608
18. X. Huang, P. Liang, B. Jiang, P. Zhang, W. Yu et al., Hyperbaric oxygen potentiates diabetic wound healing by promoting fibroblast cell proliferation and endothelial cell angiogenesis. Life Sci. 259, 118246 (2020) http:/ doi.org/10.1016/j.lfs.2020.118246
19. S. Schreml, R.M. Szeimies, L. Prantl, s. Karrer, M.Landthaler et al, Oxygen in acute and chronic wound healing. Br. J. Dermatol. 163(2), 257- 268 (2010). http:/ doi.org//101111j.1365-2133.2010.09804.x
20. P.G. Rodriguez, FN. Felix, D.T. Woodley, E.K. Shim, The role of oxygen in wound healing: a review of the literature.Dermatol. Surg 34(9), 1159-1 169 (2008). http:/ doi.org/0.0111/j.1524-4725.2008.34254.x
21. C. Huang, T. Leavitt, L.R. Bayer, D.P. Orgill, Effect of negative pressure wound therapy on wound healing. Curr. Probl.Surg. 51(7), 301-331 (2014). htps://oi.or/10. 1067/j.cpsurg.2014.04.001
22. P. Agarwal, R. Kukrele, D. Sharma, Vacuum assisted closure (VAC)negative pressure wound therapy NPWT) for difficult wounds: a review. J. Clin. Orthop. Trauma 10(5), 845- -848 (2019). https://doi.org/10.16/5.jcot.2019.06.015
23. L.C. Kloth, Discussion: advanced technologies to improve wound healing: electrical stimulation, vibration therapy, and ultrasound- what is the evidence? Plast. Reconstr. Surg. 138(35), 105S- 106S (2016). https://doi.org/10. 1097/prs. 00000000002699
24. J Rajfur, M. Pasternok, K Rajfur, K. Walewicz, B. Fras et al,Efficacy of selected electrical therapies on chronic low back pain: a comparative clinical pilot study. Med. Sci. Monit.23, 85-100 (2017). https://doi.org/10.12659/msm.899461
25. M. Ashrafi, T. Alonso-Rasgado, M. Baguneid, A. Bayat, The efficacy of electrical stimulation in lower extremity cutaneous wound healing: a systematic review. Exp. Dermatol. 26(2), 171-178 (2017). https://doi.org/10.1111/exd.13179
26. M. Norouzi, S.M. Boroujeni, N. Omidvarkordshouli, M. Soleimani, Advances in skin regeneration: application of eletrospun scaffolds. Adv. Healthcare Mater. 4(8), 1114- 1133 (2015). https://doi.org/10. 1002/adhm.201 500001
27. K. Vig, A. Chaudhari, S. Tripathi, S. Dixit, R. Sahu et al, Advances in skin regeneration using tissue engineering. Int. J. Mol. Sci. 18(4), 789 (2017). https://doi.org/0.390/jis1 8040789
28. B. Horst, G. Chouhan, NS. Moiemen, L.M Grover, Advances in keratinocyte delivery in burn wound care. Adv. Drug Deliv. Rev. 123, 18- 32 (2018). https://doi.org/10.1016/jaddr.2017. 06.012
29. Q.H. Phua, H.A. Han, B.S. Soh, Translational stem cell therapy: vascularized skin grafts in skin repair and regeneration. J. Transl. Med.19, 83 (2021). https://doi.org/10.1186/ s12967-021-02752-2
30. M.M. Martino, P.S. Briquez, A. Ranga, M.P. Lutolf, J.A. Hubbell, Heparin-binding domain of fibrin(ogen) binds growth factors and promotes tissue repair when incorporated within a synthetic matrix. PNAS 110, 4563- -4568 (2013). https://doi.org/10. 1073/pnas.1221602110
31. R. Braund, The role of topical growth factors in chronic wounds. Curr. Drug Deliv. 4(3), 195- 204 (2007). https://doi.org/10.2174/156720107781023857
32. W. Wang, KJ. Lu, C.H Yu, QL Huang, Y.Z Du, Nano drug delivery systems in wound treatment and skin regeneration. J. Nanobiotechnol. 17, 82 (2019). https://doi.org/10.1 186/ s12951-019-0514-y
33. J. Lei, L. Sun, P. Li, C. Zhu, Z. Lin et al, The wound dressings and their applications in wound healing and management. Health Sci. J.13(4), 662 (2019). https://doi.org/10. 36648/1791-809X.1000662
34. R.F. Pereira, C.C. Barrias, P.L. Granja, PJ. Bartolo, Advanced biofabrication strategies for skin regeneration and repair. Nanomedicine 8(4), 603- -621 (2013). https://doi.org/ 10.2217/m.13.50
35. R.C. Veld, X.F. Walboomers, JA. Jansen, F Wagener, Design considerations for hydrogel wound dressings: strategic and molecular advances. Tissue Eng. Part B 26(3), 230 -248 (2020).https://doi.org/10. 1089/ten.TEB.2019.0281
36. M.J. Peters, G. Stinstra, M. Hendriks, Estimation of the electrical conductivity of human tissue. Electromagnetics 21, 545- -557 (2001). https://doi.org/10. 1080/027263401752246 199
37. C. Korupalli, H. Li, N. Nguyen, FL. Mi, Y. Chang et al, Conductive materials for healing wounds: their incorporation in electroactive wound dressings, characterization, and perspectives. Adv. Healthcare Mater. 10(6), 2001384 (2020). https://doi.org/10.1002/adhm.202001384
38. TH. Qazi, R. Rai, A.R. Boccaccini, Tissue engineering of electrically responsive tissues using polyaniline based polymers: a review. Biomaterials 35(33), 9068 9086 (2014). .https://doi.org/10. 1016/j.biomaterials.2014.07.020
39. C.D. McCaig, A.M Rajnicek, B. Song, M. Zhao, Controlling cell behavior electrically: current views and future potential. Physiol. Rev. 85(3), 943- 978 (2005). https://doi.org/0.1102/21 physrev.00020.2004
40. M. Zhao, Electrical fields in wound healing-An overriding signal that directs cell migration. Semin. Cell Dev. Biol. 20(6), 674- -682 (2009). https://doi.org/10. 1016/j.semedb.2008.12.009
41. M. Zhao, B. Song, J. Pu, T. Wada, B. Reid et al, Electrical signals control wound healing through phosphatidylinositol-3-OH kinase-gamma and PTEN. Nature 442, 457- 460 (2006).https://doi.org/10. 1038/nature04925
42. Y. Wang, M. Rouabhia, D. Lavertu, Z. Zhang, Pulsed electrical stimulation modulates fibroblasts' behaviour through the Smad signalling pathway. J. Tissue Eng. Regener. Med. 11(4), 1110-1121 (2017). https://doi.org/10.1002/term.2014
43. J.C. Ojingwa, R.R. Isseroff, Electrical stimulation of wound healing. J. Invest. Dermatol. 121, 1-12 (2003). https://doi.org/10.1046/j.1523-1747.2003.12454.x
44. G. Thakral, J. LaFontaine, B. Najafi, TK. Talal, P. Kim et al, Electrical stimulation to accelerate wound healing. Diabetic Foot Ankle 4(1), 22081 (2013).https://doi.org/10.3402/dfa.v4i0.22081
45. S.S. Park, H. Kim, L.R.S. Makin, J.B. Skiba, MJ. Izadjoo, Measurement of microelectric potentials in a bioelectrically-active wound care device in the presence of bacteria. J.Wound Care 24(1), 23- -33 (2015). https://doi.org/10.12968/8jowc.2015.24.1.23
46. G. Torkaman, Electrical stimulation of wound healing: a review of animal experimental evidence. Adv. Wound Care 3(2), 202- -218 (2014). https://doi.org/10. 1089/wound.2012. 0409
47. D. Lala, SJ. Spaulding, S.M. Burke, P.E. Houghton, Electrical stimulation therapy for the treatment of pressure ulcers in individuals with spinal cord injury: a systematic review and meta-analysis. Int. Wound J. 13(6), 1214 -1226 (2016). https://doi.org/101111/iwj.12446
48. C. Khouri, s. Kotzki, M Roustit, S. Blaise, F. Gueyffier et al, Hierarchical evaluation of electrical stimulation protocols for chronic wound healing: an effect size meta-analysis. Wound Repair Regen. 25(5), 883-891 (2017).https://doi.org/10. 11/wr.12594
49. s. Ud-Din, A. Bayat, Electrical stimulation and cutaneous wound healing: a review of clinical evidence. Healthcare 2(4), 445- 467 (2014). https://doi.org/10.3390/healthcare 2040445
50. Y. Lu, Y. Wang, J. Zhang, X. Hu, Z. Yang et al, In-situ doping of a conductive hydrogel with low protein absorption and bacterial adhesion for electrical stimulation of chronic wounds. Acta Biomater. 89, 217- 226 (2019). https://doi.org/10.1016/j.actbio.2019.03.018
51. R. Dong, P.X. Ma, B. Guo, Conductive biomaterials for muscle tissue engineering. Biomaterials 229, 119584 (2020). https://doi.org/10. 1016/j.biomaterials.2019.1 19584
52. R. Balint, NJ. Cassidy, S.H. Cartmell, Conductive polymers:towards a smart biomaterial for tissue engineering. Acta Biomater, 10(6), 2341-2353 (2014). https://doi.org/10.1016/j. actbio.2014.02.015
53. TS. Sreeprasad, V. Berry, How do the electrical properties of graphene change with its functionalization? Small 9(3), 341-350 (2013). https://doi.org/10. 1002/smll.201202196
54. Q. Xin, H. Shah, A. Nawaz, w. Xie, M.Z. Akram et al, Anti- bacterial carbon-based nanomaterials. Adv. Mater.31(45), 1804838 (2019). htps://doi.org/10. 1002/adma.201 804838
55. A.G. MacDiarmid, Synthetic metals: a novel role for oganic polymers. Synth. Met. 125(1), 11- 22 (2001). https://doi.org/ 10.1016/S0379-6779(01 )00508-2
56. B. Guo, L. Glavas, A.C. Albertsson, Biodegradable and electrically conducting polymers for biomedical applications. Prog. Polym. Sci. 38(9), 1263-1286 (2013). https://doi.org/10.1016/j.progpolymsci.2013.06.003
57. R. Urie, D. Ghosh, I. Ridha, K. Rege, Inorganic nanomaterials for soft tissue repair and regeneration. Annu. Rev. Biomed. Eng. 20, 353- -374 (2018). https://doi.org/10.1 146/ annurev-bioeng-071516-044457
58. M. Kaushik, R. Niranjan, R. Thangam, B. Madhan, V. Pandiyarasan et al, Investigations on the antimicrobial activity and wound healing potential of ZnO nanoparticles. Appl. Surf. Sci. 479, 1169-1177 (2019). https://doi.org/0.1016/j.apsusc. 2019.02.189
59. M. Wu, Z. Zhang, Z. Liu, J. Zhang, Y. Zhang et al, Piezoelectric nanocomposites for sonodynamic bacterial elimination and wound healing. Nano Today 37, 101 104 (2021).https://doi.org/10.1016/j.nantod.2021.101104
60. C.Y. Chen, H. Yin, X. Chen, T.H. Chen, H.M. Liu et al., Angstrom-scale silver particle- embedded carbomer gel pro- motes wound healing by inhibiting bacterial colonization and inflammation. Sci. Adv. 6, eaba0942 (2020). https://doi.org/ 10.1126/sciadv.aba0942
61. M. Talikowska, X. Fu, G. Lisak, Application of conducting polymers to wound care and skin tissue engineering: a review. Biosens. Bioelectron. 135 50 -63 (2019). https://doi.org/10. 1016/j.bios.2019.04.001
62. R.K. Mohammad, A.A. Abdurahman, MA. Dar, M.O. Aijaz, M.L. Mollah et al., Conducting and biopolymer based electrospun nanofiber membranes for wound healing applications.Curr. Nanosci. 12(2), 220-227 (2016).https://doi.org/10. 2174/157341371 1666150415003655
63. G.S. Hussey, J.L. Dziki, SF. Badylak, Extracellular matrixbased materials for regenerative medicine. Nat. Rev. Mater. 3, 159- 173 (2018) https://doi.org/10.1038/841578-018-0023-x
64. G.S. Schultz, A. Wysocki, Interactions between extracellular matrix and growth factors in wound healing. Wound Repair Regen. 17(2), 153-162 (2009). https://doi.org/0.1111/j1 1524-475X.2009.00466.x
65. M.P. Nikolova, M.S. Chavali, Recent advances in biomaterials for 3D scaffolds: a review. Bioact. Mater. 4, 271-292 (2019). https://doi.org/10.0 1016/j.bioactmat.2019.10.005
66. G.D. Mogosanu, A.M. Grumezescu, Natural and synthetic polymers for wounds and burns dressing. Int. J. Pharm. 463(2), 127-136 (2014). https://doi.org/. 1016/j.ijpharm. 2013.12.015
67. M. Mir, M.N. Ali, A. Barakullah, A. Gulzar, M. Arshad et al., Synthetic polymeric biomaterials for wound healing: a review. Prog. Biomater. 7, 1-21 (2018). https://doi.org/10. 1007/s40204-018-0083-4
68. M. Okamoto, B. John, Synthetic biopolymer nanocomposites for tissue engineering scaffolds Prog. Polym. Sci 38(10- 11), 1487-1503 (2013). https://doi.org/ l0.1016/j.progpolymsci. 2013.06.001
69. H. Tian, Z. Tang, X. Zhuang, X. Chen, X. Jing, Biodegradable synthetic polymers: preparation, functionalization and biomedical application. Prog. Polym. Sci. 37(2), 237- -280 (2012). https://doi.org/10.1016/j.progpolymsci.201 1.06.004
70. LD. Koh, J. Yeo, YY Lee, Q. Ong, M Han et al, Advancing the frontiers of silk fibroin protein-based materials for futuristic electronics and clinical wound-healing (Invited review). Mater. Sci. Eng. C 86, 151-172 (2018). https://doi.org/10. 1016/j.msec.201 8.01.007
71. B. Guo, P.X. Ma, Synthetic biodegradable functional polymers for tissue engineering: a brief review. Sci. China Chem. 57, 490- 500 (2014). https://doi. org/ 10.1007/ s1 1426-014-5086-y
72. J. Boateng, O. Catanzano, Advanced therapeutic dressings for effective wound healing- -a review. J. Pharm. Sci. 104(11), 3653- -3680 (2015). https://doi.org/10. 1002/jps.24610
73. Y. Liang, J. He, B. Guo, Functional hydrogels as wound dressing to enhance wound healing. ACS Nano 15(8), 12687- 12722 (2021). htps://doi.org/10.102 1/acsnano.1c04206
74. Y. Liu, s. Zhou, Y. Gao, Y. Zhai, Electrospun nanofibers as a wound dressing for treating diabetic foot ulcer. Asian J. Pharm. Sci. 14(2), 130-143 (2019). htps://oi.org/10.1016/j. ajps.2018.04.004
75. Z. Liu, X. Zhang, S. Poyraz, S.P. Surwade, S.K. Manohar, Oxidative template for conducting polymer nanoclips. J Am. Chem. Soc. 132(38), 13158-13159 (2010). https:/doi.org/0. 1021/ja105966c
76. Z. Zha, X. Yue, Q. Ren, Z. Dai, Uniform polypyrrole nano- particles with high photothermal conversion efficiency for photothermal ablation of cancer cells. Adv. Mater. 25(5), 777-782 (2013). https:/doi.org/10.1002/adma.201202211
77. E.N. Zare, P. Makvandi, B. Ashtari, F. Rossi, A. Motahari et al., Progress in conductive polyaniline-based nanocomposites for biomedical applications: a review. J. Med. Chem. 63(1), 1-22 (2020). https://doi.org/10. 1021/acs.jmedchem. 9b00803
78. X. Xiao, G.R. Chen, A. Libanori, J. Chen, Wearable tribo-electric nanogenerators for therapeutics. Trends Chem.3(4), 279- 290 (2021). https:/doi.org/10.1016/j.trechm.2021.01.
79. B. Guo, P.X. Ma, Conducting polymers for tissue engineering, Biomacromol 19(6), 1764-1782 (2018). https:/doi.org/
10.1021/acs.biomac.8b00276
80. S. Nambiar, JT. Yeow, Conductive polymer-based sensors for biomedical applications. Biosens. Bioelectron. 26(5), 1825-1832 (201 1). https:/doi.org/10./ 101 6/j.bios.2010.09. 046
81. Y. Xu, Z. Shi, X. Shi, K. Zhang, H. Zhang, Recent progress in black phosphorus and black phosphorus-analogue materials: properties, synthesis and applications. Nanoscale 11(31), 14491-14527 (2019). https:/doi.org/10.1039/C9NR04348A
82. C.0. Baker, X. Huang, W. Nelson, R.B. Kaner, Polyaniline nanofibers: broadening applications for conducting polymers. Chem. Soc. Rev. 46(5), 1510 -1525 (2017). https:/doi.org//0.1039/C6CS00555A
83. K. Guo, DL. Zhang, XM. Zhang, J. Zhang, L.S. Ding et al,Conductive elastomers with autonomic self-healing properties. Angew. Chem. Int. Ed. 54(41), 12127-12133 (2015).htts://doi.org/10. 1002/anie.201 505790
84. G. Cai, J. Wang, K. Qian, J. Chen, s. Li et al, Extremely stretchable strain sensors based on conductive self-healing dynamic cross-links hydrogels for human-motion detection. Adv. Sci. 4(2), 1600190 (2017). https:/doi.org/10.1002/advs. 201600190
85. E.L. Hopley, S. Salmasi, D.M. Kalaskar, A.M. Seifalian, Carbon nanotubes leading the way forward in new generation 3D tissue engineering. Biotechnol. Adv. 32(5), 1000- -1014 (2014). https:/doi.org/10.0 1016/j.biotechadv.2014.05.003
86. G. Moku, V.R. Gopalsamuthiram, T.R. Hoye, J. Panyam, Surface modification of nanoparticles: methods and applications. Srurface Modification of Polymers (Wiley-VCH) chapter 11,317- -346 (2019). https:/doi.org/ 10.1002/97835278 19249.ch11
87. P. Humpolicek, V. KaSparkova, J. Pachernik, J. Stejskal, P.Bober et al, The biocompatibility of polyaniline and polypyr-role: a comparative study of their cytotoxicity, embryotoxicity and impurity profile. Mater. Sci. Eng. C 91, 303- -310 (2018). https:/doi.org/10 1016/j.msec.2018.05.037
88. G. Jia, H. Wang, L. Yan, X. Wang, R. Pei et al., Cytotoxicity of carbon nanomaterials: single- wall nanotube, multi-wall nanotube, and fullerene. Environ. Sci. Technol. 39, 1378-1383 (2005). https:/doi.org/10.1021/es0487291
89. Y. Liu, K. Xu, Q Chang, M.A. Darabi, B. Lin et al, Highly flexible and resilient elastin hybrid cryogels with shape memory, injectability, conductivity, and magnetic responsive prop-erties. Adv. Mater. 28(35), 7758 -7767 (2016). https:/doi.org/10.1002/adma.201 601066
90. SJ. Song, LS. Raja, Y.B. Lee, M.S. Kang, HJ. Seo et al,Comparison of cytotoxicity of black phosphorus nanosheets in different types of fibroblasts. Biomater. Res. 23, 23 (2019). https:/doi.org/10.1186/s40824-019-0174-x
91. D. An, J. Fu, Z. Xie, C. Xing, B. Zhang et al, Progress in the therapeutic applications of polymer-decorated black phosphorus and black phosphorus analog nanomaterials in biomedicine. J. Mater. Chem. B 8(32), 7076- -7120 (2020). https:/doi.org/10. 1039/D0TB00824A
92. M. Soleymaniha, M.A. Shahbazi, A.R. Rafeerad, A. Maleki,A. Amiri, Promoting role of MXene nanosheets in biomedical sciences: therapeutic and biosensing innovations. Adv.Healthcare Mater. 8(1), 1801137 (2019). https:/doi.org/10.0 1002/adhm.201801 137
93. R. Huang, X. Chen, Y. Dong, X Zhang, Y, Wei et al, MXene composite nanofbers for cell culture and tissue engineering. ACS Appl. Bio Mater. 3(4), 2125 -2131 (2020). https:/doi.org/10. 1021/acsabm.0c00007
94. S. Xiong, X. Chen, Y. Liu, T. Fan, Q. Wang et al, Black phosphorus as a versatile nanoplatform: from unique properties to biomedical applications. J. Innov. Opt. Health Sci. 13, 2030008 (2020). https:/doi.org/10.1 142/s17935458203000
95. T. Habib, X. Zhao, S.A. Shah, Y. Chen, W. Sun et al, Oxidation stability of TizCTx MXene nanosheets in solvents and composite films. npj 2D Mater. Appl. 3, 8 (2019). https:/doi.org/10.1038/s41699-019-0089-3
96. H. Xu, L. Ma, H. Shi, C. Gao, C. Han, Chitosan -hyaluronie acid hybrid film as a novel wound dressing: in vitro and in vivo studies. Polym. Adv. Technol.18(11), 869- -875
(2007). https:/doi.org/10. 1002/pat.906
97. D. Aycan, B. Selmi, E Kelel, T. Yildirim, N. Alemdar, Conductive polymeric film loaded with ibuprofen as a wound dressing material. Eur. Polym. J. 121, 109308 (2019) https:// doi.org/10.1016/j.eurpolymj.2019. 109308
98. D.D. Ateh, P. Vadgama, H.A. Navsaria, Culture of human keratinocytes on polypyrrole-based conducting polymers. Tissue Eng. 12(4), 645- -655 (2006). https:/doi.org/10.0108/9/ ten.2006.12.645
99. LJ. Valle, D. Aradilla, R. Oliver, F. Sepulcre, A. Gamez et al, Cellular adhesion and proliferation on poly(3,4-eth- ylenedioxythiophene); benefits in the electroactivity of the conducting polymer. Eur. Polym. J. 43(6), 2342- 2349 (2007). https:/doi.org/10.1016/j.eurpolymj.2007.03.050
100. R. Deepachitra, V. Ramnath, T.P. Sastry, Graphene oxide incorporated collagen-fibrin biofilm as a wound dressing material. RSC Adv. 4(107), 62717- 62727 (2014). https:// doi.org/10. 1039/C4RA 10150B
101. G, Shi, M. Rouabhia, S. Meng, Z. Zhang, Eletrical stimulation enhances viability of human cutaneous fibroblasts on conductive biodegradable substrates. J, Biomed. Mater. Res. Part A 84A(4), 1026-1037 (2008). https:/doi.org/10.1002/ jbm.a.31337
102. L. Groenendaal, F. Jonas, D. Freitag, H. Pielartzik, J.R. Reynolds, Poly(3,4-ethylenedioxythiophene) and its derivatives: past, present, and future. Adv. Mater. 12(7), 481- 494
(2000).https:/doi.org/10. 1002/(SIC)1521-4095200004) 12:7%3c48 :AID-ADMA481%3e3.0.CO;2-C
103. J.Y. Lee, C.E. Schmidt, Amine-functionalized polypyrrole: inherently cell adhesive conducting polymer. J. Biomed. Mater. Res. Part A 103(6), 2126- 2132 (2015). https://doi.org/10.1002/jbm.a.35344
104. J.H. Collier, JP. Camp, T.W. Hudson, C.E. Schmidt, Syn- thesis and characterization of polypyrrole- hyaluronic acid composite biomaterials for tissue engineering applications. J. Biomed. Mater. Res. 50(4), 574 -584 (2000). htps://oi.org/10.1002/(SIC)1097-4636(20000615)50:4%3c574:AID- JBM13%3e3.0.CO;2-I
105. K. Svennersten, MH. Bolin, E.W.H. Jager, M. Berggren, A. Richter- Dahlfors, Electrochemical modulation of epithelia formation using conducting polymers. Biomaterials 30(31), 6257- -6264 (2009). https:/doi.org/10. 101 6/j. biomaterials. 2009.07.059
106. A. Akkouch, G. Shi, Z. Zhang. M. Rouabhia, Bioactivating electrically conducting polypyrrole with fibronectin and bovine serum albumin. J. Biomed. Mater. Res. Part A 92A(1), 221- -231 (2010). https:/doi.org/10. 1002/jbm.a.32357
107. A.M. Wan, DJ Brooks, A. Gumus, C. Fischbach, G.G. Malliaras, Electrical control of cell density gradients on a conducting polymer surface. Chem. Commun. 279(35), 5278- -5280 (2009). https:/doi.org/10. 1039/b911130a
108. A. Gumus, JP. Califano, A.M.D. Wan, J. Huynh, C.A. Rein- hart-King et al, Control of cell migration using a conducting polymer device. Soft Matter 6(20), 5138- -5142 (2010).https:/doi.org/1/0 1039/B923064E
109. E.M. Stewart, M. Fabretto, M. Mueller, PJ. Molino, HJ. Griesser et al., Cell attachment and proliferation on high conductivity PEDOT- glycol composites produced by vapour phase polymerisation. Biomater. Sci. 1(4), 368- 378 (2013). https:/doi.org/10. 1039/C2BM00143H
110. S.K. Yadav, Y.C. Jung, JH. Kim, YI. Ko, HJ. Ryu et al, .Electrically conductive biocomposite films using antimicrobial chitosan-functionalized graphenes. Part. Part. Syst. Charact. 30, 721-727 (2013). https:/doi.org/0 1002/ppsc. 201 300044
111. J, Banerjee, P. DasGhatak, s. Roy, S. Khanna, E.K. Sequin et al., Improvement of human keratinocyte migration by a redox active bioelectric dressing. PLoS ONE 9, e89239 (2014). https:/doi.org/10.137/1 1/journal.pone 0089239
112. M. Marzocchi, I. Gualandi, M. Calienni, I. Zironi, E. Scavetta et al., Physical and electrochemical properties of PEDOT:PSS as a tool for contolling cell growth. ACS Appl. Mater. Inter- faces 7(32), 17993-18003 (2015). https:/doi.org/10.1021/ acsami.5b04768
113. NA. Chowdhury, A.M. Al-Jumaily, Regenerated cellulose/ polyprrole/silver nanoparticlesionic liquid composite films for potential wound healing applications. Wound Med.14, 16-18 (2016). https:/doi.org/10. 1016/j.wndm.2016.07.001
114. N. Pramanik, K. Dutta, RK. Basu, PP. Kundu, Aromatic π-conjugated curcumin on surface modified polyaniline/ polyhydroxyalkanoate based 3D porous scaffolds for tissue engineering applications. ACS Biomater. Sci. Eng. 2(12), 2365- 2377 (2016). https:/doi.org/10. 1021/acsbiomaterials. 6b00595
115. S.H. Bhang, W.S. Jang, J. Han, JK. Yoon, W.G. La et al,Zinc oxide nanorod-based piezoelectric dermal patch for wound healing. Adv. Funct. Mater. 27(1), 1603497 (2017).
https:/doi.org/ 10.1002/adfm.201603497
116. Y. Long, H. Wei,J, Li, G. Yao, B. Yu et al, Effective wound healing enabled by discrete alternative eletric fields from wearable nanogenerators. ACS Nano 12(12), 12533-12540 (2018).https:/doi.org/10. 1021/acsnano.8b07038
117. M. Khamrai, S.L. Banerjee, S. Paul, AK. Ghosh, P. Sarkar et al, A mussel mimetic, bioadhesive, antimicrobial patch based on dopamine-modified bacterial cellulose/rGO/Ag NPs: a green approach toward wound-healing applications. ACS Sustain. Chem. Eng. 7(14), 12083- -12097 (2019). https:/doi.org/10. 102 1/acssuschemeng9b01163
118. M. Li,J. Chen, M. Shi, H. Zhang, PX. Ma et al, Electroactive anti-oxidant polyurethane elastomers with shape memory property as non-adherent wound dressing to enhance wound healing. Chem. Eng. J. 375, 121999 (2019) https:/doi.org/10.1016/j.cej.2019.121999
119. X. Fu, JK. Wang, A.C. Ramirez-Perez, C. Choong, G. Lisak, Flexible conducting polymer-based cellulose substrates for on- skin applications. Mater. Sci Eng C 108, 110392 (2020). https:/doi.org/10.1016/j.msec.2019.110392,
120. s. Liu, J. Li, s. Zhang, X. Zhang, J, Ma et al, Template- assisted magnetron sputtering of cotton nonwovens for wound healing application. ACS Appl. Bio Mater. 3(2), 848 -858 (2020). https:/doi.org/10.0 1021/acsabm.9b00942
121. L. Zhang, Y. Yu, s. Zheng. L. Zhong, J. Xue, Preparation and properties of conductive bacterial cellulose based graphene oxide-silver nanoparticles antibacterial dressing. Carbohydr. Polym. 257, 117671 (2021). https:/doi.org/10.1016/j.carbp ol.2021.117671
122. X.W. Huang, J. Wei, M.Y. Zhang, X.L. Zhang, XF. Yin . et al., Water-based black phosphorus hybrid nanosheets as a moldable platform for wound healing applications. ACS Appl. Mater. Interfaces 10(41), 35495 35502 (2018). https:/doi.org/10.1021/acsami.8b12523
123. w. Wentao, Z. Tao, s. Bulei, Z. Tongchang, Z. Qicheng et al, Functionalization of polyvinyl alcohol composite film wrapped in am-ZnO@CuO@ Au nanoparticles for antibacterial application and wound healing. Appl. Mater. Today 17, 36- 44 (2019). https:/doi.org/10.1016/j.apmt.2019.07.001
124. TJ. Simmons, S.H. Lee, TJ. Park, D.P. Hashim, PM. Ajayan et al, Antiseptic single wall carbon nanotube bandages. Carbon 47(6), 1561-1564 (2009). https:/doi.org/10.1016/j.carbon.2009.02.005
125. TJ. Simmons, CJ. Rivet, G, Singh, J. Beaudet, E. Sterner et al, Application of carbon nanotubes to wound healing biotechnology. in Nanomaterials for Biomedicine (ACS Symposium Series) chapter 7, 155- 174 (2012). https:/doi.org/0.0 1021/bk-2012-1119.ch007
126. G. Shi, Z. Zhang, M. Rouabhia, The regulation of cell functions electrically using biodegradable polypyrrole- polylactide conductors. Biomaterials 29(28), 3792 -3798 (2008).
https:/doi.org/10.1016/j.biomaterials.008.06.010
127. M. Rouabhia, H. Park, s. Meng, H. Derbali, Z. Zhang, Electrical stimulation promotes wound healing by enhancing dermal fibroblast activity and promoting myofibroblast transdifferentiation. PLoS ONE 8, e71660 (2013). https:/doi.org/0.1371/journal.pone.0071660
128. HJ. Park, M. Rouabhia, D. Lavertu, Z. Zhang, Electrical stimulation modulates the expression of multiple wound healing genes in primary human dermal fibroblasts. Tissue Eng. Part A 21(13-14), 1982-1990 (2015) https:/doi.org/ 10.1089/ten.TEA.2014.0687
129. C.L. Weaver, J.M. LaRosa, x. Luo, X.T. Cui, Electrically controlled drug delivery from graphene oxide nanocomposite films. ACS Nano 8(2), 1834- 1843 (2014). https:/doi.org/0. 1021/nn406223e
130. G.A. Justin, S. Zhu, T.R. Nicholson, J. Maskrod, J. Mbugua et al, On- demand controlled release of anti-inflammatory and analgesic drugs from conducting polymer films to aid in wound healing. in 34th Annual International Conference of the IEEE EMBS, San Diego, California USA, (28 August - 1 September, 2012). https:/doi.org/10.1 109/EMBC.2012.6346153
131. T.M. Nguyen, S. Lee, S.B. Lee, Conductive polymer nanotube patch for fast and controlled ex vivo transdermal drug delivery. Nanomedicine 9(15), 2263 -2272 (2014). https:/doi.org/10.2217/0nm.13.153
132. B. Guo, A. Finne-Wistrand, A.C. Albertsson, Facile synthesis of degradable and electrically conductive polysaccharide hydrogels. Biomacromol 12(7), 2601 -2609 (201 1). https:// doi.org/10.1021/bm200389t
133. A. Masotti, M.R. Miller, A. Celluzzi, L. Rose, F. Micciulla et al, Regulation of angiogenesis through the efficient delivery of microRNAs into endothelial cells using polyamine- coated carbon nanotubes. Nanomedicine 12(6), 1511-1522 (2016). https:/doi.org//.01/j.nano.2016.02.0170
134. W. Yang, Y. Wang, J. Li, X. Yang, Polymer wrapping technique: an effective route to prepare Pt nanoflower/carbon nanotube hybrids and application in oxygen reduction. Energ. Environ. Sci. 3(1), 144 149 (2010). https:/doi.org/doior/0.10390/ B916423E
135. S. Wei, X. Zou, J. Tian, H Huang, w. Guo et al, Control of protein conformation and orientation on graphene. J. Am.Chem. Soc. 141(51), 20335- -20343 (2019). https:/doi.org/10.1021/jacs .9b10705
136. S. Shahmoradi, H. Golzar, M. Hashemi, V. Mansouri, M.Omidi et al, Optimizing the nanostructure of graphene oxide/silver/arginine for efective wound healing. Nanotechnology 29(47), 475101 (2018). https:/doi.org/10.1088/1361-6528/aadedc
137. H. Kai, T. Yamauchi, Y. Ogawa, A. Tsubota, T. Magomeet al, Accelerated wound healing on skin by electrical stim- ulation with a bioelectric plaster. Adv. Healthcare Mater. 6(22), 1700465 (2017). https:/doi.org/0.1002/adhn.201700465
138. J. Shao, C. Ruan, H. Xie, Z. Li, H. Wang et al, Black-phos-phorus-incorporated hydrogel as a sprayable and biodegrad- able photothermal platform for postsurgical treatment of cancer. Adv. Sci. 5(5), 1700848 (2018). https:/doi.org/10. 1002/advs.201 700848
139. P. Zhang, B. Sun, F. Wu, Q. Zhang, X. Chu et al, Wound healing acceleration by antibacterial biodegradable black phosphorus nanosheets loaded with cationic carbon dots. J, Mater. Sci. 56, 6411- 6426 (2021).https:/doi.org/10.1007/ s10853-020-05766- 1
140. C. Xue, L. Sutrisno, M. Li, w. Zhu, Y. Fei et al, Implantable multifunctional black phosphorus nanoformulation-depos-ited biodegradable scaffold for combinational photothermal/ chemotherapy and wound healing. Biomaterials 269, 120623 (2021). https:/doi.org/10. 1016/j.biomaterials.2020.120623
141. L. Mao, S. Hu, Y. Gao, L. Wang, W. Zhao et al, Biodegrad- able and electroactive regenerated bacterial cellulose/MXene (TizC2T) composite hydrogel as wound dressing for acceler- ating skin wound healing under electrical stimulation. Adv. Healthcare Mater. 9(19), 2000872 (2020) https:/doi.org/10.1002/adhm.202000872
142. E.A. Mayerberger, R.M. Street, R.M. McDaniel, M.W. Bar-soum, CL. Schauer, Antibacterial properties of electrospun TizC2T2 (MXene)/chitosan nanofibers. RSC Adv. 8(62), 35386- -35394 (2018). https:/doi.org/10.1039/C8RA06274A
143. L. Zhou, H. Zheng, Z Liu, s. Wang, Z. Liu et al., Conductive antibacterial hemostatic multifunctional scaffolds based on TijC2Tx MXene nanosheets for promoting mulidug-resistant bacteria-infected wound healing. ACS Nano 15(2), 2468-2480 (2021). https:/doi.org/10.1021/acsnano.0c06287
144. M.S. Pacheco, G.E. Kano, LA. Paulo, P.S. Lopes, M.A. de Moraes, Silk fibroin/chitosan/alginate multilayer membranes as a system for controlled drug release in wound healing. Int. J. Biol. Macromol. 152, 803- 811 (2020). https:/doi.org/10.0 1016/j. ijbiomac.2020.02.140
145. E.A. Kamoun, E.R.S. Kenawy, X. Chen, A review on poly-meric hydrogel membranes for wound dressing applications: PVA-based hydrogel dressings. J. Adv. Res. 8(3), 217-233 (2017). https:/doi.org/10.0 1016/j.jare.2017.01.005
146. R. Gharibi, H. Yeganeh, H. Gholami, ZM. Hassan, Aniline tetramer embedded polyurethane/siloxane membranes and their corresponding nanosilver composites as intelligent wound dressing materials. RSC Adv. 4(107), 62046 -62060(2014). https:/doi.org/10.1039/04RA11414J44
147. M. Alexandru, M. Cazacu, M. Cristea, A. Nistor, C. Grigoras et al, Poly(siloxane-urethane) crosslinked structures obtained by sol-gel technique. J. Polym. Sci. Part A Polym. Chem. 49(7), 1708 -1718 (201 1). https:/doi.org/10.002/pola.24602
148. R. Gharibi, H. Yeganeh, A.R. Lactoee, ZM. Hassan, Stimulation of wound healing by elctroactive, antibacterial, and antioxidant polyurethane/siloxane dressing membranes: in vitro and in vivo evaluations. ACS Appl. Mater. Interfaces 7(43), 24296- -24311 (2015). https:/doi.org/10.1021/acsami. 5b08376
149. Y.C. Hsiao, P.R. Jheng, HT. Nguyen, Y.H. Chen, YB. Manga et al, Photothermal iradiated polyethyleneimine-polypyrrole nanopigment film-coated polyethylene fabrics for infrared- inspired with pathogenic evaluation. ACS Appl. Mater. Interfaces 13(2), 2483- -2495 (2021). https:/doi.org/10.1021/1 acsami.0c17169
150. F. Croisier, G. Atanasova, Y. Poumay, C. Jerome, Polysaccha-ride-coated PCL nanofibers for wound dressing applications.Adv. Healthcare Mater. 3(12), 2032- 2039 (2014).https:/doi.org/10.1002/adhm.201400380
151. S.I Jeong, L.D. Jun, MJ. Choi, Y.C. Nho, Y.M. Lee et al, Development of electroactive and elastic nanofibers that contain polyaniline and poly(L-lactide-co-e-caprolactone) for the control of cell adhesion. Macromol. Biosci. 8(7), 627- 637 (2008). https:/doi.org/10. 1002/mabi.200800005
152. M. Gizdavic-Nikolaidis, S. Ray, J.R. Bennett, AJ. Easteal, R.P. Cooney, Electrospun functionalized polyaniline copolymer-based nanofibers with potential application in tissue engineering. Macromol. Biosci. 10(12), 1424 1431 (2010). htts://doi.org/10. 1002/mabi.201000237
153. S. Aznar-Cervantes, M.L Roca, J.G. Martinez, L. Meseguer- Olmo, JIL. Cenis et al, Fabrication of conductive electrospun silk fibroin scaffolds by coating with polypyrrole for biomedical applications. Bioelectrochemistry 85, 36- 43 (2012). https:/doi.org/10.101/.biocechem.2011.1 1.008
154. B. Lu, T. Li, H. Zhao, X. Li, C. Gao et al, Graphene based composite materials beneficial to wound healing. Nanoscale 4(9), 2978- 2982 (2012).https:/doi.org/10 1039/C2NR1 1958G
155. Y. Wang, M. Roubhia, Z. Zhang, PPy-coated PET fabrics and electric pulse-stimulated fibroblasts. J. Mater. Chem. B 1(31), 3789 -3796 (2013). https:/doi.org//./ 1039/C3TB2 0257G
156. X. Niu, M. Rouabhia, N. Chiffot, M.W. King, Z Zhang, An electrically conductive 3D scaffold based on a nonwoven web of poly(-lactic acid) and conductive poly(3,4-ethylenedioxy- thiophene). J. Biomed. Mater. Res. Part A 103(8), 2635- 2644 (2015). https:/doi.org/10.1002/jb0.354058088
157. Y. Wang, M. Rouabhia, Z. Zhang, Pulsed electrical stimulation benefits wound healing by activating skin fibroblasts through the TGFB1/ERKNF-KB axis. Biochim. Biophys.Acta 1860(7), 1551-1559 (2016). https:/doi.org/10.1016/j bbagen.2016.03.023
158. H.C. Chang, T. Sun, N. Sultana, M.M. Lim, T.H. Khan et al., Conductive PEDOT:PSS coated polylactide (PLA) and poly(3-hydroxybutyrate-co-3-hydroxyvalerate) (PHBV) electrospun membranes: fabrication and characterization. Mater. Sci. Eng. C 61, 396- 410 (2016). https:/doi.org/0./
1016/jmsec.2015.12.074
159. HK. Patra, Y. Sharma, M.M. Islam, MJ. Jafari, N.A. Murugan et al., Inflammation sensitive in situ smart scaffolding for regenerative medicine. Nanoscale 8(39), 17213- 17222 (2016). https:/doi.org/10. 1039/C6NR06157E
160. G. Ruiz-Velasco, F. Martinez-Flores, J. Morales-Corona, R. Olayo-Valles, R. Olayo, Polymeric scaffolds for skin. Macromol. Symp. 374(1), 1600133 (2017). https:/doi.org/10.1002/masy.201600133
161. D. Gh, D. Kong, J. Gautrot, S.K. Vootla, Fabrication and characterization of conductive conjugated polymer coated Antheraea mylitta silk fibroin fibers for biomedical applications. Macromol. Biosci. 17(7), 1600443 (2017). https:/doi.org/10. 1002/mabi.201600443
162. Q. Zhang, Q. Du, Y. Zhao, F. Chen, Z. Wang et al, Graphene oxide-modified electrospun polyvinyl alcohol nanofibrous scaffolds with potential as skin wound dressings. RSC Adv.
7(46), 28826 28836 (2017). https:/doi.org/10.1039/C7RA0 3997B
163. B.K. Gu, SJ. Park, C.H. Kim, Beneficial effict of aligned nanofiber scaffolds with electrical conductivity for the directional guide of cells. J. Biomater. Sci. Polym. Ed. 29(7 -9),
1053- -1065 (2018). https:/doi.org/10.1080/09205063.2017. 1364097
164. R. Roman-Doval, M.M. Tellez-Cruz. H. Rojas-Chavez, H. Cruz-Martinez, G. Carrasco-Torres et al, Enhancing electrospun scaffolds of PVP with polypyrrole/iodine for tissue engineering of skin regeneration by coating via a plasma process. J. Mater. Sci. 54, 3342 -3353 (2019). https:/doi.org/10.1007/s10853-018-3024-7
165. J. He, Y. Liang, M. Shi, B. Guo, Anti-oxidant electroactive and antibacterial nanofibrous wound dressings based on poly(e-caprolactone /quaternized chitosan-graft-polyaniline
for full-thickness skin wound healing. Chem. Eng. J.385, 123464 (2020). https:/doi.org/0.016/0j.201.9.123414144
166. M. Zarei, A. Samimi, M. Khorram, M.M. Abdi, S.I. Golestaneh, Fabrication and characterization of conductive polypyrrole/chitosan/collagen electrospun nanofiber scaffold for tissue engineering application. Int. J. Biol. Macromol.168, 175-186 (2021). https:/doi.org/g/ 10.101 6/j.ijbiomac. 2020.12.031
167. I. Altinbasak, R. Jijie, A. Barras, B. Golba, R. Sanyal et al, Reduced graphene-oxide-embedded polymeric nanofiber mats: an“on-demand" photothermally triggered antibiotic release platform. ACS Appl. Mater. Interfaces 10(48), 41098- 41 106 (2018) https:/doi.org/10. 1021/acsami.8b1478
168. Z. Jia, J. Gong, Y. Zeng, J. Ran, J. Liu et al, Bioinspired conductive silk microfiber integrated bioelectronic for diagnosis and wound healing in diabetes. Adv. Funct. Mater. 31(19),2010461 (2021). htps://doi.org/10. 1002/adfm.202010461
169. B.W. Walker, R.P. Lara, E. Mogadam, C.H. Yu, W. Kimball et al, Rational design of microfabricated electroconductive hydrogels for biomedical applications. Prog. Polym. Sci 92, 135- 157 (2019).https:/doi.org/10. 101 6/j.progpolymsci.2019.02.007
170. J. Xu, Y.L. Tsai, S.H. Hsu, Design strategies of conductive hydrogel for biomedical applications. Molecules 25(22), 5296 (2020). https:/doi.org/10.339/0/molecules25225296
171. J. Qu, X. Zhao, Y. Liang, T Zhang, P.X. Ma et al, Antibacterial adhesive injectable hydrogels with rapid self-healing, extensibility and compressibility as wound dressing for joints skin wound healing. Biomaterials 183, 185- -199 (2018). https:/doi.org/10.1016/j.biomaterials.2018.08.044
172. Y. Liang, Z. Li, Y. Huang, R. Yu, B. Guo, Dual-dynamicbond CrOss-linked antibacterial adhesive hydrogel sealants with on-demand removability for post-wound-closure and
infected wound healing. ACS Nano 15(4), 7078 -7093 (2021).https://doi.org/10. 1021/acsnano.1c00204
173. R. Yu, Y. Yang, J. He, M. Li, B. Guo, Novel supramolecular self-healing silk fibroin-based hydrogel via host- guest interaction as wound dressing to enhance wound healing. Chem.Eng. J. 417, 128278 (2021). https:/doi.org/10. 1016/j.cej.2020.128278
174. X. Zhao, H. Wu, B. Guo, R. Dong, Y. Qiu et al, Antibacterial anti- oxidant eletroactive injectable hydrogel as self-healing wound dressing with hemostasis and adhesiveness for cutaneous wound healing. Biomaterials 122.34 47 (2017). https:// doi.org/10. 1016/j.biomaterials.2017.01.011
175. M. di Luca, O. Vittorio, G, Cirillo, M. Curcio, M. Czuban et al, Electro-responsive graphene oxide hydrogels for skin bandages: the outcome of gelatin and trypsin immobilization. Int. J. Pharm. 546(1- 2), 50- 60 (2018). https:/doi.org/j.ijpharm.2018.05.027
176. D. Gan, L. Han, M. Wang, W. Xing, T. Xu ct al, Conductive and tough hydrogels based on biopolymer molecular templates for controlling in situ formation of polypyrrole nanorods. ACS Appl. Mater. Interfaces 10(42), 36218- 36228 (2018). https:/doi.org/10.0 1021/acsami8b10280
177. C. Mao, Y. Xiang, X. Liu, z. Cui, X. Yang et al, Repeatable photodynamic therapy with triggered signaling pathways of fibroblast cell proliferation and differentiation to promote bacteria-accompanied wound healing. ACS Nano 12(2), 1747-1759 (2018). https:/doi.org/./ 1021/acsnano.7b08500
178. M.K. Satapathy, B. Nyambat, C.W. Chiang, C.H. Chen, P.C. Wong et al., A gelatin hydrogel-containing nano organic PEI Ppy with a photothermal responsive eftct for tssue engineering applications. Molecules 23(6), 1256 (2018). https:/doi.org/10.3390/molecules23061256
179. Y. Liang, X. Zhao, T. Hu, B. Chen, Z. Yin et al, Adhesive hemostatic conducting injectable composite bydrogels with sustained drug release and photothermal antibacterial activity to promote fll-thickness skin regeneration during wound healing. Small 15(12), 1900046 (2019). https:/doi.org//..1002/smll.201900046
180. Y. Liang, X. Zhao, T. Hu, Y. Han, B. Guo, Mussel inspired,antibacterial, conductive, antioxidant, injectable composite hydrogel wound dressing to promote the regeneration of infected skin. J. Colloid Interface Sci. 556, 514- -528 (2019). https:/doi.org/10.1016/jjcis.2019.08.083
181. J. Qu, X. Zhao, Y. Liang, Y. Xu, P.X. Ma et al., Degradable conductive injectable hydrogels as novel antibacterial, anti- oxidant wound dressings for wound healing. Chem. Eng. J. 362, 548- -560 (2019). https:/doi.org/10. 1016/j.cej.2019.01.028
182. Y. Zhao, Z. Li, s. Song, K. Yang, H. Liu et al, Skin-inspired antibacterial conductive hydrogels for epidermal sensors and diabetic foot wound dressings. Adv. Funct. Mater. 29(31), 1901474 (2019). https:/doi.org/10.1002/adfm.201901474
183. J He, M. Shi, Y. Liang, B. Guo, Conductive adhesive self-healing nanocomposite hydrogel wound dressing for photothermal therapy of infected ful-thickness skin wounds. Chem. Eng. J. 394, 124888 (2020). https:/doi.org/10.1016/j.0 cej.2020.12488
184. X. Jin, Y. Shang, Y. Zou, M. Xiao, H. Huang et al., Injectable hypoxia-induced conductive hydrogel to promote diabetic wound healing. ACS Appl. Mater. Interfaces 12(51), 56681- -56691 (2020). https:/doi.org/10. 1021/acsami.0c131
185. M. Li, Y. Liang, J. He, H. Zhang, B. Guo, Two-pronged strategy of biomechanically active and biochemically multifunctional hydrogel wound dressing to accelerate wound closure and wound healing. Chem. Mater. 32(23), 9937- 9953 (2020). https:/doi.org/10. 1021/acs.chemmater.0c02823
186. S. Li, L. Wang, w. Zheng, G. Yang, X. Jiang, Rapid fabrication of self-healing, conductive, and injectable gel as dressings for healing wounds in stretchable parts of the body. Adv.Funct. Mater. 30(31), 2002370 (2020).https:/doi.org/10. 1002/adfm.202002370
187. Y. Liang, B. Chen, M. Li,J. He, Z. Yin et al, Injectable antimicrobial conductive hydrogels for wound disinfection and infectious wound healing. Biomacromol 21(5), 1841-1852 (2020). https:/doi.org/10. 1021/acs.biomac 9b01732
188. J. Ouyang, x. Ji, X. Zhang, C. Feng, Z. Tang et al, In situ sprayed NIR-responsive, analgesic black phosphorus-based gel for diabetic ulcer treatment. PNAS 117, 28667- -28677 (2020). https:/doi.org/10.1073/as.2016268117
189. B. Zhang, J. He, M. Shi, Y. Liang, B. Guo, Injectable selfhealing supramolecular hydrogels with conductivity and photo-thermal antibacterial activity to enbance complete skin regeneration. Chem. Eng. J.400, 125994 (2020).https:/doi.org/10.1016/j.cej.2020.125994
190. J. Zhang, C. Wu, Y. Xu, J. Chen, N. Ning et al, Highly stretchable and conductive self-healing hydrogels for temperature and strain sensing and chronic wound treatment. ACS Appl. Mater. Interfaces 12(37), 40990 40999 (2020).https:/doi.org/10.102 1/acsami.0c08291
191. X. Zhang, G. Chen, Y. Liu, L. Sun, L. Sun et al., Black phosphorus-loaded separable microneedles as responsive oxygen delivery carriers for wound healing. ACS Nano 14(5), 5901-5908 (2020). https:/doi.org/0.1021/acsnano.0c01059
192. Y. Zhao, Z. Li, Q. Li, L. Yang, H. Liu et al, Transparent conductive supramolecular hydrogels with stimuli-responsive properties for on-demand dissolvable diabetic foot wound dressings. Macromol. Rapid Commun. 41(24), 2000441 (2020). https:/doi.org/10 1002/marc.202000441
193. Y. Zhu, J. Zhang, J. Song. J. Yang, Z. Du et al., A multifunctional pro-healing zwittrionic hydrogel for simultaneous optical monitoring of pH and glucose in diabetic wound treatment. Adv. Funct. Mater. 30(6), 1905493 (2020). https:/doi.org/10.1002/adfm.201905493
194. S.H. Jeong, Y. Lee, M.G. Lee, WJ. Song et al, Accelerated wound healing with an ionic patch assisted by a triboelectrie nanogenerator. Nano Energy 79, 105463 (2021). https:/doi.org/10.1016/j.nanoen.2020. 105463
195. Z. Li, w. Xu, x. Wang, W. Jiang, X. Ma et al., Fabracation of PVA/PAAm IPN hydrogel with high adhesion and enhanced mechanical properties for body sensors and antibacterial activity. Eur. Polym. J. 146, 110253 (2021).https:/doi.org/ 10.1016/j.eurpolymj.2020.1 10253
196. C. Hu, L. Long,J. Cao, S. Zhang, Y. Wang, Dual- crosslinked mussel-inspired smart hydrogels with enhanced antibacterial and angiogenic properties for chronic infected diabetic wound treatment via pH-responsive quick cargo release. Chem. Eng. J.411, 128564 (2021). https:/doi.org/10.1016/j.cej.2021.128564
197. Y. Wang, Y. Lu, J. Zhang, X. Hu, Z. Yang et al, A syner-gistic antibacterial effect between terbium ions and reduced graphene oxide in a poly(vinyl alcohol)- -alginate hydrogel for treating infected chronic wounds. J. Mater Chem. B 7(4), 538- -547 (2019).https:/doi.org/10.1039/C8TB02679C
198. Z. Fan, B. Liu, J. Wang, S. Zhang, Q Lin et al, A novel wound dressing based on Ag/graphene polymer hydrogel: effectively kill bacteria and accelerate wound healing. Adv. Funct. Mater.24(25), 3933- -3943 (2014). https://doi.org/10. 1002/adfm.201304202
199. S.O. Blacklow, J. Li, B.R. Freedman, M. Zeidi, C. Chen et al, Bioinspired mechanically active adhesive dressings to accelerate wound closure. Sci. Adv. 5(7), eaaw3963 (2019). https:/doi.org/10.1126/ciadv.aaw3963
200. L. Yan, T. Zhou, L, Han, M. Zhu, Z. Cheng et al, Conductive cellulose bio -nanosheets assembled biostable hydrogel for reliable bioelectronics. Adv. Funct. Mater. 31(17), 2010465 (2021). https:/doi.org/10 1002/adfm.202010465
201. C.W. Hsiao, HL. Chen, Z.X. Liao, R. Sureshbabu, H.C. Hsiao et al., Effective photothermal killing of pathogenic bacteria by using spatially tunable colloidal gels with nanolocalized heating sources. Adv. Funct. Mater. 25(5), 721-728 (2015). https:/doi.org/10 1002/adfm.201403478
202. H.B.T. Moran, JL. Turley, M. Andersson, E.C. Lavelle, Immunomodulatory properties of chitosan polymers. Biomaterials 184, 1-9 (2018). https:/doi.org/10.1016/j.biomaterials.2018.08.054
203. X. Zhao, P. Li, B. Guo, P.X. Ma, Antibacterial and conductive injectable hydrogels based on quaternized chitosan-graft-polyaniline/oxidized dextran for tissue engineering. Acta Biomater. 26, 236- 248 (2015). https:/doi.org//0/ 1016/j.actbio.2015.08.006
204. A. Ramadan, M. Elsaidy, R. Zyada, Effect of low-intensity direct current on the healing of chronic wounds: a literature review. J. Wound Care 17(7), 292- -296 (2008). https:/doi.org//10. 12968/jowc.2008.17.7.30520
205. Z.Li, H. Wang, B. Yang, Y. Sun, R. Huo, Three-dimensional graphene foams loaded with bone marrow derived mesenchymal stem cells promote skin wound healing with reduced scarring. Mater. Sci. Eng. C 57, 181-188 (2015). https:/doi.org/10.1016/j.msec.2015.07.062
206. C. Nie, G. Zhang, D. Yang, T. Liu, D. Liu et al, Targeted delivery of adipose-derived stem cells via acellular dermal matrix enhances wound repair in diabetic rats. J. Tissue Eng. Regener, Med. 9(3), 224 -235 (2015). https:/doi.org/10.1002/term.1622
207. Q. Wang, Y. Jin, X. Deng, H. Liu, H. Pang et al, Second-har-monic generation microscopy for assessment of mesenchymal stem cell-seeded acellular dermal matrix in wound-healing. Biomaterials 53, 659 -668 (2015). https:/doi.org/10.101/1.1 biomaterials.2015.03.01 1
208. J. Rnjak- Kovacina, A.S. Weiss, Increasing the pore size of clectrospun scaffolds. Tissuc Eng. Part B 17(5), 365- 372 (201 1). https:/doi.org/10.0 1089/ten.teb.2011.0235
209. X. Zhang, D. Chang,J. Liu, Y. Luo, Conducting polymer aerogels from supercritical CO, drying PEDOT-PSS hydrogels. J. Mater. Chem. 20(24), 5080- -5085 (2010).https:/doi.org/10.1039/C0JM00050G
210. G.R. Mueller, TJ. Pineda, HX. Xie, J.S Teach, A.D. Barof- sky et al, A novel sponge-based wound stasis dressing to treat lethal noncompressible hemorrhage. J. Trauma Acute Care Surg. 73(2), S134 S139 (2012). https:/doi.org/10.1097/1TA.0b013e3182617c3c
211. JL.S. Antonio, L.M. Lira, V.R. Goncales, S.I.C. Torresi, Fully conducting hydro- sponges with electro-swelling properties tuned by synthetie parameters. Electrochim. Acta 101, 216- 224 (2013). https:/doi.org/10. 1016/.electacta.2012.11.012
212. Y. Lu, W. He, T. Cao, H. Guo, Y. Zhang et al, Elastic, conductive, polymeric hydrogels and sponges. Sci. Rep. 4, 5792(2014). https:/doi.org/10. 1038/srep05792
213. H. Maleki, L. Duraes, C.A. Garcia-Gonzalez, P. Gaudio, A.Portugal et al, Synthesis and biomedical applications of aerogels: Possibilities and challenges. Adv. Colloid Interface Sci. 236, 1-27 (2016). https:/doi.org/10.1016/j.cis.2016.05.01 1
214. D.M. Supp, S.T. Boyce, Engineered skin substitutes: practices and potentials. Clin. Dermatol. 23(4), 403- 412 (2005).https:/doi.org/10. 1016/j.clindermatol.2004.07.023
215. A.D. Metcalfe, M.WJ. Ferguson, Bioengineering skin using mechanisms of regeneration and repair. Biomaterials 28(34), 5100- -5113 (2007). https:/doi.org/10.1016/j.biomaterials. 2007 .07.031
216. M.L. lorio, J. Shuck, C.E. Attinger, Wound healing in the upper and lower extremities: a systematic review on the use of acellular dermal matrices. Plast. Reconstr. Surg. 130,232S-241S (2012). https:/doi.org/10.1097/PRS.0b013e3182615703
217. X. Guo, D. Mu, F. Gao, Eficacy and safety of acellular dermal matrix in diabetic foot ulcer treatment: a systematic review and meta- analysis. Int. J. Surg.40, 1-7 (2017). https:/doi.org/10.1016/j.jsu.2017.02.008
218. S. Zhou, M. Wang, X. Chen, F. Xu, Facile template synthesis of microfibrillated cellulose/polyprrole/silver nanoparticles hybrid aerogels with electrical conductive and pressure responsive properties. ACS Sustain. Chem. Eng. 3(12),3346- 3354 (2015). https:/doi.org/0.1021/acsschemeng.5b01020
219. P. Thangavel, R. Kannan, B. Ramachandran, G. Moorthy,L. Suguna et al, Development of reduced graphene oxide (rGO)-isabgol nanocomposite dressings for enhanced vascularization and accelerated wound healing in normal and diabetic rats. J. Colloid Interface Sci. 517, 251-264 (2018). https://doi.org/10.1016/j.jcis.2018.01.110
220. M.R. Santos, JJ. Alcaraz- Espinoza, M.M. Costa, H.P. Oliveira, Usnic acid-loaded polyaniline/polyurethane foam wound dressing: preparation and bactericidal activity. Mater. Sci. Eng C 89, 33 40 (2018). https:/doi.org/10.101/j.ie1c0.2018.03.019
221. X. Zhao, B. Guo, H. Wu, Y. Liang, P.X. Ma, Injectable antibacterial conductive nanocomposite cryogels with rapid shape recovery for noncompressible hemorrhage and wound healing. Nat. Commun. 9, 2784 (2018). https:/doi.org/10. 1038/s4 1467-018-04998-9
222. FA.G. Silva, C.M.S. Araljo, J. Alcaraz-Espinoza, HP. Oliveira, Toward flexible and antibacterial piezoresistive porous devices for wound dressing and motion detectors. J. Polym. Sci. Part B Polym. Phys. 56(14), 1063- -1072 (2018). https://doi.org/10. 1002/polb.24626
223. J Fu, Y Zhang, J. Chu, X. Wang, W. Yan et al, Reduced graphene oxide incorporated acellular dermal composite scaffold enables eficient local delivery of mesenchymal stem cells for accelerating diabetic wound healing. ACS Biomater. Sci. Eng. 5(8), 4054- 4066 (2019). https:/doi.org/10.0 1021/acsbi omaterials. 9b00485
224. R. Ma, Y. Wang, H Qi, C. Shi, G. Wei et al, Nanocomposite sponges of sodium alginate/graphene oxide/polyvinyl alcohol as potential wound dressing: in vitro and in vivo evaluation. Compos. B Eng. 167, 396- 405 (2019). https:/doi.org/10. 1016/j.compositesb.2019.03.006
225. P. Tang, L. Han, P. Li, Z. Jia, K. Wang et al, Mussel-inspired electroactive and antioxidative scafolds with incorporation of polydopamine-reduced graphene oxide for enhancing skin wound healing. ACS Appl. Mater, Interfaces 11(8), 7703- 7714 (2019). https:/doi.org/0.1021/acsami.8b18931
226. Y. Chen, Y. Liang, J. Liu, J. Yang, N. Jia et al, Optimizing microenvironment by integrating negative pressure and exogenous electric fields via a flexible porous conductive dressing to accelerate wound healing. Biomater. Sci. 9(1), 238 -251 (2021). https://doi.org/10.1039/D0BM01172J
227. R. Shechter, M. Schwartz, CNS sterile injury: just another wound healing? Trends Mol. Med. 19(3), 135-143 (2013). https:/doi.org//0./ 1016/j.molmed.2012.11.007
228. P. Zhang, B. Zou, Y.C. Liou, C. Huang, The pathogenesis and diagnosis of sepsis post burn injury. Burns Trauma 9, tkaa0477 (2021). https:/doi.org/10. l093/burns/tkaa047
229. R. Nunan, K.G. Harding, P. Martin, Clinical challenges of chronic wounds: searching for an optimal animal model to recapitulate their complexity. Dis. Model. Mech. 7(11), 1205-1213 (2014).https:/doi.org/0 1242/dmm.016782
230. A.A. Chaudhari, K. Vig, D.R. Baganizi, R. Sahu, s. Dixit et al, Future prospects for scaffolding methods and biomaterials in skin tissue engineering: a review. Int. J. Mol. Sci. 17(12), 1974 (2016). https:/doi.org/10.3390/jms17121974
231. S. Guo, L.A. DiPietro, Factors affecting wound healing. J. Dent. Res. 89(3), 219- 229 (2010). https:/doi.org/10.1177/ 0022034509359125
232. A. McLister, J McHugh, J. Cundell, J. Davis, New develop- ments in smart bandage technologies for wound diagnostics. Adv. Mater. 28(27), 5732- -5737 (2016). https:/doi.org/10. 1002/adma.201504829
233. NX. Landen, D. Li, M. Stahle, Transition from infammation to proliferation: a critical step during wound healing. Cell. Mol. Life Sci. 73, 3861- -3885 (2016).https:/doi.org/10.1007/ s00018-016-2268-0
234. T. Nezakati, A. Seifalian, A. Tan, A.M. Seifalian, Conductive polymers: opportunities and challenges in biomedical applications. Chem. Rev. 118(14), 6766 6843 (2018). https:/doi.org/10. 1021/acs.chemrev.6b00275
235. C. Chen, X. Bai, Y. Ding, I.S. Lee, Electrical stimulaion as a novel tool for regulating cell behavior in tissue engineering. Biomater. Res. 23, 25 (2019).https:/doi.org/10.1 186/ s40824-019-0176-8
236. Q. Gao, X. Zhang, W. Yin, D. Ma, C. Xie et al, Functionalized MoS2 nanovehicle with near-infrared laser-mediated nitric oxide release and photothermal activities for advanced bacteria-infected wound therapy. Small 14(45), 1802290 (2018). https:/doi.org/10.0 002/m1.201 802290
237. Q. Shi, X. Luo, Z. Huang, A.C. Midgley, B. Wang et al, Cobalt- mediated multi-functional dressings promote bacteria-infected wound healing. Acta Biomater. 86, 465- 479 (2019). https:/doi.org/10.106/j.actbio.2018.12.048
238. M.A. Shahbazi, M.P. Ferreira, H.A. Santos, Landing a lethal blow on bacterial infections: an emerging advance of nanodots for wound healing acceleration. Nanomedicine 14, 2269- 2272 (2019). https:/doi.org/10.221/nm-2019-0236
239. Q. Pang, D. Lou, S. Li, G. Wang, B. Qiao et al, Smart flexible electronics- integrated wound dressing for real-time monitoring and on demand treatment of infected wounds. Adv. Sci. 7(6), 1902673 (2020). https:/doi.org/0.1002/dvs.20190 2673
240. C. Korupalli, C.C. Huang, W.C. Lin, W.Y. Pan, P.Y. Lin et al, Acidity-triggered charge-convertible nanoparticles that can cause bacterium-specific aggregation in situ to enhance photothermal ablation of focal infection. Biomaterials 116, 1-9 (2017). https:/doi.org/10. 1016/j.biomaterials.2016.11.045
241. X. Dai, Y. Zhao, Y. Yu, X. Chen, X. Wei et al., Single continuous near-infrared laser-triggered photodynamic and photothermal ablation of antibiotic-resistant bacteria using effetive targeted copper sulfide nanoclusters. ACS Appl. Mater. Interfaces 9(36), 30470- 30479 (2017). https:/doi.org/10. 102 1/acsami.7b09638
242. X. Yang, P. Xia, Y. Zhang. S. Lian, H. Li et al, Photothermal nano-antibiotic for efective treatment of mulidrug-resistant bacterial infection. ACS Appl. Bio Mater. 3(8), 5395- -5406 (2020). https:/doi.org/1/0./ 1021/acsabm.0c00702
243. WL. Chiang, TT Lin, R. Sureshbabu, WT. Chia, H.C. Hsiao et al., A rapid drug release system with a NIR light-activated molecular switch for dual-modality photothermal/antibiotic treatments of subcutaneous abscesses. J. Control, Release 199, 53- 62 (2015). https:/doi.org/ 10.1016j jconrel.2014. 12.011
244. L. Zhao, L. Niu, H. Liang, H. Tan, C. Liu et al, pH and glucose dual-responsive injectable hydrogels with insulin and fibroblasts as bioactive dressings for diabetic wound healing. ACS Appl. Mater. Interfaces 9(43), 37563- -37574 (2017). https:/doi.org/10.102 1/acsami.7b09395
245. H. Nosrati, R.A. Khouy, A. Nosrati, M. Khodaei, M. Banitalebi-Dehkordi et al,, Nanocomposite scaffolds for accelerating chronic wound healing by enhancing angiogenesis.
J. Nanobiotechnology 19, 1 (2021). https:/doi.org/1/.0/ 186/s12951-020-00755-7
246. A. Kumar, T. Behl, S. Chadha, A rationalized and innovative perspective of nanotechnology and nanobiotechnology in chronic wound management. J. Drug Delivery Sci. Technol. 60, 101930 (2020). https:/doi.org/10.1016/jjddst.2020. 101930
247. R. Dong, B. Guo, Smart wound dressings for wound healing.Nano Today 41, 101290 (2021). https:/doi.org/10.1016/j.nantod.2021.101290
248. Z. Deng, R. Yu, B. Guo, Stimuli-responsive conductive hydrogels: design, properties, and applications. Mater. Chem. Front. 5(5), 2092- -2123 (2021). https://doi.org/10. 1039/ D0QM00868K
249. R. Rahimi, M. Ochoa, T. Parupudi, X. Zhao, IK. Yazdi et al, A low-cost flexible pH sensor array for wound assessment. Sens. Actuat. B 229, 609- -617 (2016).https:/doi.org//0./ 1016/j.snb.2015.12.082
250. L. Lipani, B.G.R. Dupont, F. Doungmene, F. Marken, R.M.Tyrrell et al, Non-invasive, transdermal, path-selective and specific glucose monitoring via a graphene-based platform. Nat. Nanotechnol.13, 504- 511 (2018). https://doi.org/10. 1038/s41565-018-0112-4
251. R. Barber, S. Cameron, A. Devine, A. McCombe, L. Kirsty Pourshahidi et al., Laser induced graphene sensors for assessing pH: application to wound management. Electrochem. Commun.123, 106914 (2021). https:/doi.org/10. 1016/j. elecom.2020.106914
252. A. Tamayol, M. Akbari, Y. Zilberman, M. Comotto, E. Lesha et al.. Flexible pH-sensing hydrogel fibers for epidermal applications. Adv. Healthcare Mater. 5(6), 711-719 (2016). https://doi.org/10. 1002/adhm.201500553
253. L.A. Schneider, A. Korber, S. Grabbe, J. Dissemond, Infiuence of pH on wound healing: a new perspective for wound-therapy? Arch. Dermatol. Res. 298 413- 420 (2007). https:/doi.org/10.1007/s00403-006-0713-x
254. T. Guinovart, G. Valdes-Ramirez, J.R. Windmiller, FJ.Andrade, J. Wang, Bandage- based wearable potentiometric sensor for monitoring wound pH. Electroanalysis 26(6),1345-1353 (2014). https:/doi.org/0.1002/elan.20130058
255. P. Mostafalu, A. Tamayol, R. Rahimi, M. Ochoa, A. Khalil-pour et al, Smart bandage for monitoring and treatment of chronic wounds. Small 14(33), 1703509 (2018). https:/doi.org10.1002/smll.201703509
256. S.D. Milne, I. Seoudi, HA. Hamad, TK. Tallal, A.A. Anoop et al., A wearable wound moisture sensor as an indicator for wound dressing change: an observational study of wound moisture and status. Int. WoundJ. 13(6), 1309-1314 (2016). https:/doi.org/101111/iwj.12521
257. S. RoyChoudhury, Y. Umasankar, J. Jaller, I. Herskovitz, J. Mervis et al, Continuous monitoring of wound healing using a wearable enzymatic urie acid biosensor. J. Electrochem. Soc. 165, B3168 -B3175 (2018).https:/doi.org/10.1149/2.2 0231808jes
258. X. Zhang, J. Chen, J. He, Y. Bai, H. Zeng, Mussel-inspired adhesive and conductive hydrogel with tunable mechanical properties for wearable strain sensors. J. Colloid Interface Sci. 585, 420- 432 (2021). https:/doi.org/10.1016/.jcis.2020. 10.023
259. T. Someya, Z. Bao, G.G. Malliaras, The rise of plastic bioelectronics. Nature 540, 379 -385 (2016). https:/doi.org/0. 1038/nature21004
260. M. Jia, M. Rolandi, Soft and ion-conducting materials in bioelectronics: from conducting polymers to hydrogels. Adv. Healthcare Mater. 9(5), 1901372 (2020). https:/doi.org/g/10.1002/adhm.201901372
261. P. Chansai, A. Sirivat, S. Niamlang. D. Chotpattananont, K.Viravaidya-Pasuwat, Controlled transdermal iontophoresis of sulfsalicylic acid from polypyrrole/poly(acrylic acid) hydrogel. Int. J. Pharm. 381(1), 25- -33 (2009). https://doi.org/10.1016/.jpharm.2009.07.019
262. X. Luo, XT. Cui, Sponge-like nanostructured conducting polymers for electrically controlled drug release. Electrochem. Commun. 11(10), 1956- -1959 (2009). https:/doi.org/10.1016/j elecom.2009.08.027
263. N. Paradee, A. Sirivat, Electrically controlled release of benzoic acid from poly(3,4-ethylenedioxythiophene)/alginate matrix: effect of conductive poly(3,4-ethylenedioxythio- phene) morphology. J. Phys. Chem B 118(31), 9263- -9271 (2014). https:/doi.org/10. 1021/jp502674f
264. C.J. Perez-Martinez, S.D.M. Chavez, T. Castillo-Castro,TEL. Ceniceros, M.M. Castillo-Ortega et al, Electrocon-ductive nanocomposite hydrogel for pulsatile drug release.React, Funct. Polym.100, 12- -17 (2016). https://doi.org/10.1016/j.reactfunctpolym.2015.12.017
265. S. Niamlang. N. Paradee, A. Sirivat, Hybrid transdermal drug delivery patch made from poly(p phenylene vinylene)/natural rubber latex and controlled by an electric field. Polym. Int.67(6), 747- -754 (2018). https://doi.org/10.1002/i.5566
266. J. Qu, x. Zhao, P.X. Ma, B. Guo, Injectable antibacterial conductive hydrogels wih dual response to an electric field and pH for localized“smart" drug release. Acta Biomater. 72, 55- 69 (2018). https://doi.org/1/0.0 1016/j.actbio.2018.03.018
267. CF. Hsu, L Zhang, H. Peng, J. Travas Sejdic, PA. Kilmartin,Scavenging of DPPH free radicals by polyprrole powders of varying levels of overoxidation and/or reduction. Synth. Met. 158(21-24), 946952 (2008). https://doi.org/10. 1016/j.synth met.2008.06.017
268. R.L. Thangapazham, s. Sharad, R.K. Maheshwari, Skin regenerative potentials of curcumin. BioFactors 39(1), 141-149 (2013). hts://doi.org/10. 1002/biof.1078
269. P. Zarrintaj, A.S. Moghaddam, S. Manouchehri, Z. Atoufi, A.Amiri et al, Can regenerative medicine and nanotechnology combine to heal wounds? The search for the ideal wound dressing. Nanomedicine 12, 2403- 2422 (2017). https://doi. org/10.2217/nnm-2017-0173
270. Z. Su, H. Ma, Z. Wu, H. Zeng, Z. Li et al., Enhancement of skin wound healing with decellularized scaffolds loaded with hyaluronic acid and epidermal growth factor. Mater, Sci. Eng. C 44, 440- 448 (2014). https://doi.org/10. 1016/j.msec.2014.07.039
271. H. Brem, A. Kodra, M.S. Golinko, H. Entero, O. Stojadinovic et al, Mechanism of sustained release of vascular endothelial growth factor in accelerating experimental diabetic healing. J.
Invest. Dermatol.129(9), 2275- 2287 (2009) https://doi.org//
10.1038/jid.2009.26
272. N. Gomez, C.E. Schmidt, Nerve growth factor immobilized polypyrrole: bioactive electrically conducting polymer for enhanced neurite extension. J. Biomed. Mater. Res. A 81,135- -149 (2007). https://doi.org/10. 1002/jbm.a31047
273. B. Guo, J. Qu, X. Zhao, M. Zhang, Degradable conductive self-healing hydrogels based on dextran- graft-tetraaniline and N-carboxyethyl chitosan as injectable carriers for myoblast cell therapy and muscle regeneration. Acta Biomater. 84,180- -193 (2019). https://doi.org/10.1016/j.actbio.2018.12.008
274. JL. Liao, S. Zhong, S.H. Wang, J.Y. Liu, J. Chen et al, Preparation and properties of a novel carbon nanotubes/poly(vinyl alcobol)/epidermal growth factor composite biological dressing. Exp. Ther. Med.14, 2341- -2348 (2017). htps://doi.org/103892/etm.2017.4752
275. Y. Zhu, Q Zeng, Q. Zhang, K. Li, X. Shi et al, Temperature/near-infrared light-responsive conductive hydrogels for controlled drug release and real-time monitoring. Nanoscale 12(16), 8679- -8686 (2020). htps://doi.org/10. 1039/D0NR0 1736A
This article is excerpted from the Nano-Micro Letters by Wound World.
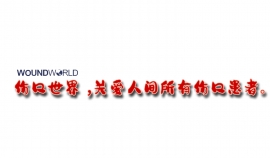
- 星期一, 19 9月 2022
Conductive Biomaterials as Bioactive Wound Dressing for Wound Healing and Skin Tissue Engineering(2)
4. 3D Conductive Biomaterials for Wound Healing
2D conductive biomaterials have made great achievement in wound healing. Through adopting multiple functions, they can promote cell attachment, proliferation, differentiation, and further the whole wound healing process. However, they are still restrained on thin and superficial wounds. In contrast, 3D biomaterials such as hydrogels, foams, and sponges possessing high water absorbance capacity can deal with wounds with high exudate [67]. On the other hand, deep wounds and chronic wounds have poor regenerative capacity due to versatile mechanism, such as lack of cell sources, severe infection, limited blood supply, immunosuppression or immunodeficiency, metabolic diseases, and other environmental factors [13]. Fortunately, 3D biomaterials possessing ECM-mimicking architecture could be utilized as scaffolds for these issues, which could not only support the integrity of dermis, but also act as carriers for bioactive reagents and cells [63].
4.1 Hydrogel
Due to the porous 3D interconnected structure and highwater content, hydrogel owns a lot of advantages as wound dressing [35, 145, 169]. Hydrogel allows oxygen and water vapor to pass through, maintains a humid environment, lowers the wound temperature, and relieves pain [170]. The soft nature, flexibility, and stretchability support hydrogel being compliant with human skin under ceaseless movement [171, 172]. Moreover, hydrogel has great tolerance to integrate multiple functions, including mechanical properties and additional therapeutic effects [173]. Correspondingly, hydrogel wound dressings have attracted most intensive attention in the past few years. Recently developed conductive hydro- gel-based wound dressings are summarized in Table 3.
So far, versatile conductive hydrogels with different conductive components have been developed. In contrast to most 2D biomaterials-based wound dressings which validate their potential in wound dressing by in vitro cell culture assay, the wound healing efficacy of most conductive hydrogels has been proved both by in vitro and in vivo animal assays. However, unlike the situations of film wound dressings where electrical activities of conductive substances can be isolated to prove their effects on cellular activities, the wound healing performance is always enhanced through synergistic effects from electrical activities, antioxidant, and antibacterial activities of hydrogel-based wound dressings. In 2015, Hsiao et al. synthesized a chitosan derivate with self- doped PANI which could form colloidal gels induced by pH increase and explored the photothermal antibacterial activities in vitro and on subcutaneous abscess [201]. But the conductivity and the electroactivity were not examined in this work. Since then, these relevant features of the conductive substances were comprehensively evaluated via standard protocols. Our group has designed multifunctional conductive hydrogel-based wound dressings and proven their positive performance in accelerating wound healing process [165, 179 -181, 183, 185, 187]. Chitosan, a natural polysaccharide, has been utilized in wound dressing for a long time, owing to its inherent antibacterial, analgesic effect and hemostatic activity [202]. However, the poor solubility of chitosan under neutral and constrained antibacterial effectiveness under nonacidic environments extremely limit its application and efficacy of wound healing. Fortunately, the abundant active amino groups enable further modification. Quaternized chitosan (QCS) is a better choice for its excellent antibacterial activities and improved solubility. Our group not only solves the above issues, but also broadens its derivates and applications as wound dressing.
As shown in Fig. 8, quaternized chitosan-g-polyaniline (QCSP) synthesized and demonstrated good water solubility and enhanced antibacterial activity and biocompatibility than pure chitosan [203]. Meanwhile, the residue active amino groups remained potential to react with other groups. In this work, QCSP was then crosslinked with benzaldehyde group functionalized poly (ethylene glycol)-Co-poly (glycerol sebacate) via Schiff base forming the hydrogel network [174]. The optimal hydrogel dressing showed an ionic conductivity of 2.37 mS cm-1 that is close to that of human dermis, thus owning the ability to transfer bioelectrical signals for accelerating wound healing. Overall, com- pared with Tegaderm™ film, the optimal hydrogel dressing performed excellent enhanced wound healing covering all stages, including in vivo blood clotting capacity, promoted ECM synthesis, collagen deposition, granulation tissue thickness, and promoted remolding phase. We also developed a supramolecular conductive hydrogel based on QCS and graphene oxide graft-cyclodextrin [189]. The dynamic host-guest interactions were employed as crosslinkers endowing the hydrogel with self- healing and injectability. Considering the antibacterial activity, cell proliferation, and hemocompatibility, the hydrogel with 0.4 wt% of rGO was selected as the optimal dressing. Indeed, this conductive hydrogel dressing exhibited enhanced wound healing on full-thickness wounds. It is worthy to mention that , we validated that Pluronic F127 and polydopamine are of great advantages in designing carbon nanomaterials incorporated hydrogels, including assisting homogeneous dispersion, improving mechanical properties and tissue adhesiveness [183, 185].
In addition, conductive hydrogels can promote the efficiency of electrotherapy. Commonly in clinic, small metal electrodes were attached on human body near the wounds; thus, ES could not directly cover the whole wound [194]. As the large impedance of human skin, it is only possible to apply ES on every inch of wounds under high voltage, which may threat more danger to the patients [24, 204]. In scientific research, conductive films and fabrics have been justified to promote wound healing under ES for covering the whole wound bed [42, 115, 155, 157]. Reasonably, conductive hydrogel is another good choice, in terms of conductivity, softness, stretchability, and flexibility. Recently, a conductive hydrogel containing poly(2-hydroxyethyl methacrylate) and PPy has justified superior to commercial hydrogel dressing considering the antibacterial capacity and alleviated secondary damage during removal. Moreover, the significance of this work was that the replacement of traditional separate electrodes with one integral conductive hydrogel can extremely promote the efficacy of electrotherapy [50]. Zhang et al. created a conductive hydrogel using Zn2+ and PPy as the conductive components and chitosan as the main polymer backbone. This hydrogel was capable of sensing temperature and strain variations and accelerating the infected chronic wounds with ES [190]. More impressively, Jeong et al. developed an ionic hydrogel dressing based on LiCl and combined the dressing with a prototypical wearable triboelectric nanogenerator [194]. The nanogenerator can harvest biophysical energy from friction between skin and deliver ES to hydrogel, while the hydrogel dressing directly distributes ES to the whole wound.
Another attractive feature of hydrogel biomaterial is the great potential in tissue engineering by acting as scaffold to support cells and biomolecules. Mesenchymal stem cell combined with an ECM- mimicking biomaterial has attracted much attention in chronic wound healing [205- 207]. Conductive hydrogel has been employed as scaffold for the treatment of diabetic wounds. Jin et al. recently reported a conductive hydrogel scaffold based on AT, hyaluronic acid and gelatin [184]. Compared with nonconductive hydrogel, the conductive hydrogel was found to upregulate the level of Cx43, owing to better transport of molecules and ions between cells. What's even more impressive, O2-consuming enzyme laccase was introduced to cast a hypoxic microenvironment, and this hypoxic environment could maintain for almost 12 h. Furthermoe, adipose-derived mesenchymal stem cells were loaded for direct delivery to the hostile wound, while relative cell activity remained higher than 85% within 2 days. Thus, this conductive hydrogel could act as a multifunctional scaffold for chronic wounds. Overall, conductive hydrogels could promote wound healing process via diversiform approaches, thus being regarded as valuable candidates for wound healing, particularly for complicated chronic wounds. On the other hand, the excellent conductivity, easy fabrication method, and facile surface modification enable conductive hydrogels with great potential in health care devices for wound diagnosis. But , the long-term durability of hydrogel may impede this progress.
4.2 Fibrous Scaffold
Even though electrospun scaffolds have been reckoned as promising candidates for tissue engineering, their applications still constrained by several factors, such as pore size and pore interconnectivity that all affect cellular infiltration and tissue ingrowth into the scaffold. Small pore size did not hinder the application for nanofibers as wound dressing, but cell attachment and proliferation might be restrained on the surface of nanofibers [162, 1 64]. Nanofibers with too compact structures could not fulfill the requirements of porous scaffolds for tissue engineering applications [208]. A nonwoven conductive web composed of PEDOT and PLLA was fabricated by melt-spinning. After culture for 48 h, human dermal fibroblasts appeared throughout the scaffold, indicating the web permitted cell infiltration [156]. Another interesting work is about a polyaniline-multi-walled carbon nanotube/PNIPAm composite electrospun nanofibers-based “smart”scaffold with temperature responsiveness for cell delivery [159]. Above LCST, this conductive nanofibrous
scaffolds demonstrated enhanced fibroblast attachment and proliferation, while below LCST, the encapsulated cells would detach and been delivered to human body. Moreover, this stimuli-responsive nanofiber network was inflammation-sensitive, and can deal with loco-regional acidosis, which helps to pass through the inflammation phase. Therefore, conductive nanofibrous scaffold could be envisioned with great value in skin tissue engineering with deliberate design.
4.3 Sponge, Foam, and Acellular Dermal Matrix
Hydrogel-derived aerogel and cryogel have sponge-like structures and high polarity for water absorption and thus could not only manage with a large amount of water, but also permit water to flow out/in freely [209- 213]. Foam also has an interconnected porous structure and is commonly manufactured from polyurethane or silicone [9, 15]. Without further modifications with a hydrophilic surface, the pure foam demonstrates hydrophobicity, thus benefiting inherent antibacterial properties [33]. The subtle difference between sponge and foam is that foam usually exhibited more enhanced mechanical properties than sponge [33]. Generally, foam and sponge dressings can be used for various types of wounds, including burn, ulcer, skin donor area, and transplant. Also, they are lightweight, elastic, and easy to use in practice. As the second layer of skin, the dermis consists of a connective ECM with fibroblasts, endothelial cells, smooth muscle cells, and mast cells [214, 215]. ECM supports the main structure of skin tissue, develops interactions with versatile growth factors, and modulates cellular activities [63]. Acellular dermal matrix (ADM) derived from human or animal skin has been widely used in tissue engineering and wound healing as tissue replacement, graft and wound dressing [216, 217] The currently developed 3D conductive wound dressings are listed in Table 4.
Our group developed a conductive cryogel composed of chitosan and PF127- assisted homodispersed CNT, while CNT providing conductivity and reinforcement toward the mechanical properties, as shown in Fig. 9 [221]. Com- pared with cryogel from pure natural polymers, commercial gelatin hemostatic sponge, and Combat Gauze, this CNT hybrid cryogel (QCSG/CNT) demonstrated rapid blood- triggered shape recovery and absorption speed, high blood uptake capacity, and hemostatic capability. Moreover, CNT provided this cryogel with photothermal effect and NIR- assisted photothermal antibacterial activity. Compared with commercial Tegaderm™ film and nonconductive cryogel, the conductive cryogel demonstrated better wound healing performance with the least inflammatory infiltration, and the highest vascularization by 15 days.
The ADM comprised of extracellular matrix proteins and collagen demonstrates excellent biocompatibility, suitable mechanical properties, and bioactivity which is ideal for skin tissue scaffold. Fu et al. developed a rGO incorporated ADM-based scaffold via simple solution immersion process in which ADM was crosslinked with EDC and NHS [223]. The topology and structural integrity preserved after loading with rGO. Compared with the primitive scaffold and scaffold loaded with GO, ADM-rGO demonstrated superior cell attachment and proliferation for mesenchymal stem cells and human skin fibroblasts. Eventually, acting as a transplanting platform for mesenchymal stem ells, this conductive scaffold demonstrated enhanced therapeutic effect toward diabetic wounds. Based on the above, 3D conductive biomaterials have demonstrated encouraging results in promoting loaded with GO, ADM-rGO demonstrated superior cell attachment and proliferation for mesenchymal stem cells and human skin fibroblasts. Eventually, acting as a transplanting platform for mesenchymal stem cells, this conductive scaffold demonstrated enhanced therapeutic effect toward diabetic wounds. Based on the above, 3D conductive biomaterials have demonstrated encouraging results in promoting wound healing by working as wound dressings.
3D conductive biomaterials demonstrate promising potential in electrodes for electrotherapy and scaffolds for skin tissue engineering. Chen et al. reported an Ag nanowiresloaded foam demonstrating flexibility, enhanced conductivity, and long-term stability under physiological environment [226]. Due to the inherent antibacterial activity, good water-uptake capability, and electrical conductivity, the conductive foam could not only prevent infection and manage necrosis, but also implement annular oriented electrical field to wounds assisted by exogenous electrical fields. In the in vivo experiment on full-thickness pig skin wound, compared with control group treated with gauze, wounds treated with the conductive foam absent from exogenous electrical fields demonstrated enhanced wound healing performance for smaller wound residual area, controlled inflammation, better neovascularization, and advanced re- epithelialization. More excitingly, when applying the conductive foam with exogenous electrical fields, wounds demonstrated the most superior wound healing effect and therefore proved the great value of 3D conductive biomaterials in wound dressing, as well as their application in electrotherapy. Furthermore, the intrinsic feature of the highly porous structure enabled this conductive foam to connect with negative-pressure drainage closure device, thus simultaneously promote the wound healing process. Especially, since the structure, composition, appendages, and healing mechanism of porcine skin are closer to human skin, these results are more convincing.
5. Application of Conductive Biomaterials in Wound Healing
Regardless of different types of the wounds, the healing process occurs in a similar systematic manner including four distinct phases, as illustrated in Fig.10 [227]. Ideally, hemostasis occurs immediately after injury and would complete within seconds or hours depending on wound size, depth, and wound location. Then, inflammation begins and lasts for several days and reaches the highest level by 72 h. The third phase, proliferation is more complicated. Angiogenesis, fibroblast migration, granulation tissue formation, collagen deposition, epithelialization, and wound contraction take place simultaneously. Finally, the last remodeling phase allowing granulation tissue to develop into mature connective tissue may last for several months to years. With standard wound care, acute wounds can progress through the healing routine steadily. However, in practice, normal wound healing would be affected and disrupted by many factors, including nutrition, oxygen supply, infection, aging, chronic disease, wound treatment, and even genetics. Extensive tissue damage, necrotic debris, and diseases often make wounds suffer from such issues, thus leading to prolonged inflammation and delayed proliferation and remodeling. Wound with delayed healing more than 3 months would be referred to as chronic wound [10, 228, 229]. In detail, chronic wounds with impaired regenerative capacity demonstrate high levels of proinflammatory cytokines, persistent infections, and drug resistance. Apparently, chronic wounds need specific treatment including tissue debridement, infection clearance, moisture balance, mechanical support, and management of comorbidities according to the etiology and real-time diagnosis.
Wound dressings and skin tissue scaffolds are of great importance in wound care and skin tissue regeneration [27,160]. There have been developed plenty commercial products to fulfill the requirements for different wounds. Biomaterials with specific functions have also been symmetrically studied in promoting wound healing, such as antibacterial, hemostatic, adhesive, injectable, and antioxidant property. Conductive biomaterials demonstrate promising potential in wound healing as well, because they could regulate and promote cell attachment and relevant activities with or without ES that have been convinced by in vitro and in vivo assays [44, 61, 230]. So far, researchers have successfully validated the effectiveness of conductive biomaterials in different types of wounds, both for acute and chronic wounds. Moreover, due to the intrinsic electroconductivity, conductive wound dressings can be applied in real-time diagnosis. It is worth mentioning that conductive biomaterials are usually combined with other bioactive substances to meet the requirements in practice.
5.1 Acute Wound
An acute wound is an unintentional injury to skin that can be caused by surgical incisions, bites, deep lacerations, abrasions, and burns [2, 3, 11, 33]. Acute wounds can spontaneously heal in an orderly routine even without any external intervention. In scientific research, clean incisional and excisional wounds with controlled area and facile surgery are frequently utilized to evaluate the effectiveness of wound dressings. Generally, a full-thickness wound with clear edge is created by surgical incision on the back of rat or pig. Full- thickness wound means a loss of all layers of the skin and great potential of the exposure of underlying tissues. Deep infection and fluid exudate affect the healing process, as well [15]. To address such issues, conductive biomaterials-based wound dressings integrating multiple functions are of high needs.
5.1.1 2D Conductive Biomaterials for Acute Wound
As incisional and excisional wounds on rat or pig skin have light exudate, 2D biomaterial-based wound dressings can meet the requirements of wound care. Conductive film, membrane, and nanofibers have all realized their applications in acute full-thickness wounds. CPs and oligomers incorporated biomaterials have obtained great attention for their facile synthesis. In 2015, Gharibi et al. developed a series of polyurethane/siloxane-based conductive wound dressing containing aniline tetramer moieties [148]. These wound membranes displayed electroactivity, antimicrobial activity, and antioxidant ability which could promote fibroblast growth and proliferation. Besides, these CSA-doped membranes revealed comparable equilibrium water absorption value and water vapor transmission rate to some commercially available dressings, and suitable surface hydrophilicity to support cellular activity. Thus, the authors suggested these membranes could work as wound dressings for acute and chronic wounds, because the above three parameters are important to evaluate whether a product could maintain a moist environment for wounds. In an in vivo animal assay last for 20 days, the designed membranes exhibited accelerated wound healing than commercial cotton gauze. Our group synthesized a conductive polyurethane film, in which PCL provided mechanical properties, PEG contributed to surface wettability and AT supported electroactivity [118]. Through in vitro and in vivo assays, the conductive film with 12% AT content revealed improved cell adhesion and proliferation, and enhanced wound healing performance than nonelectroactive commercial dressing. Recently, our group also proved the viability of conductive nanofibers as wound dressing in practice. The electroactive nanofibers were electrospun from PCL and QCSP, thus demonstrating suitable mechanical properties, electroactivity and antibacterial properties [165]. The microporous structure can not only support cellular activities, but also guarantee the nanofibers to absorb exudate from wounds. The balance between antibacterial activity and cell proliferation should be taken into consideration as well, for bilateral properties of QCSP. Eventually, the conductive nanofibers with 15 wt% of QCSP were selected as the optimum dressings. Indeed, compared with Tegaderm™ film, the electroactive nanofibers exhibited improved wound healing efficiency with rapid wound contraction, higher collagen depostion, lower production of TGF-a, and higher expression of VEGF within 14 days.
Except for the antibacterial activities, metals and metal oxides can generate ES under specific conditions. Liu et al. utilized template- assisted magnetron sputtering method to modify commercial spunlace cotton nonwovens with metal dots (Ag, Zn), as shown in Fig. 11 [120]. The low content of metals enabled the dressing with good cytocompatibility. Interestingly, this conductive Ag/Zn@Cotton dressing demonstrated enhanced cell migration and accelerated wound healing, which was attributed to the generation of ES and inherent antibacterial activities of Ag t/Zn2+ with continuous release under moist conditions. Bhang et al. developed a piezoelectric dermal patch based on zinc oxide nanorod and applied this patch in treating full-thickness wounds [115]. Under small mechanical deformations, this patch generated electrical fields. In animal assay, the patch was found to promote wound healing process via a series of cellular activities, including inflammation regulation, cell proliferation, re-epithelization, angiogenic factor secretion, and tissue remodeling.
In common, to address complications in acute wounds, 2D conductive biomaterials always need to be endowed with multiple bioactive functions while fulfilling basic requirements. However, the application of 2D conductive biomaterials in acute wound healing is still restricted by some parameters, such as the limited capacity for managing exudate, loading bioactive agents and maintaining their biological activities, further functionalization, and low adhesion to skin.
The literature is to be continued……
This article is excerpted from the Nano-Micro Letters by Wound World.
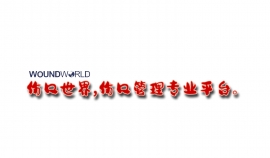
- 星期五, 16 9月 2022
Conductive Biomaterials as Bioactive Wound Dressing for Wound Healing and Skin Tissue Engineering(1)
RuiYu1, HualeiZhang1,2, BaolinGuo1,2
Baolin Guo, baoling@ mail.xjtu.edu.cn
State Key Laboratory for Mechanical Behavior of Materials, and Frontier Institute of Science and Technology, Xi' an Jiaotong University,Xi'an 710049, People's Republic of China
Key Laboratory of Shaanxi Province for Craniofacial Precision Medicine Research, College of Stomatology, Xi'an Jiaotong University,Xi'an 710049, People's Republic of China
Published online: 02 December 2021
HIGHLIGHTS
●The design and application of conductive biomaterials for wound healing are comprehensively reviewed, including versatile conductive agents, the various forms of conductive wound dressings, and different in vivo applications.
●Three main strategies of which conductive biomaterials realizing their applications in wound healing and skin tissue engineering are discussed.
●The challenges and perspectives in designing multifunctional conductive biomaterials and further clinical translation are proposed.
ABSTRACT Conductive biomaterials based on conductive polymers, carbon nanomaterials, or conductive inorganic nanomaterials demonstrate great potential in wound healing and skin tissue engineering, owing to the similar conductivity to human skin, good antioxidant and antibacterial activities, electrically controlled drug delivery, and photothermal effect. However, a review highlights the design and application of conductive biomaterials for wound healing and skin tissue engineering is lacking. In this review, the design and fabrication methods of conductive biomaterials with various structural forms including film, nanofiber, membrane, hydrogel, sponge, foam, and acellular dermal matrix for applications in wound healing and skin tissue engineering and the corresponding mechanism in promoting the healing process were summarized. The approaches that conductive biomaterials realize their great value in healing wounds via three main strategies (electrotherapy, wound dressing, and wound assessment) were reviewed. The application of conductive biomaterials as wound dressing when facing different wounds including acute wound and chronic wound (infected wound and diabetic wound) and for wound monitoring is discussed in detail. The challenges and perspectives in designing and developing multifunctional conductive biomaterials are proposed as well.
KEYWORDS Conducting polymers; Inorganic nanomaterials; Biomaterials; Electrotherapy; Wound monitoring
Cite as
Nano-Micro Lett. (2022) 14:1
Received: 29 July 2021
Acepted: 29 October 2021 ◎The Author(s) 2021
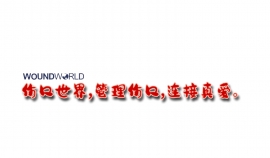
- 星期三, 14 9月 2022
应用功能材料促进皮肤创面修复
罗高兴 刘梦龙
陆军军医大学(第三军医大学)第一附属医院全军烧伤研究所,创伤、烧伤与复合伤国家重点实验室,重庆市疾病蛋白质组学重点实验室 400038
通信作者:罗高兴 Email: 该Email地址已收到反垃圾邮件插件保护。要显示它您需要在浏览器中启用JavaScript。
【摘要】皮肤创面是临床常见病症之一。功能材料通过结构调节和性能整合,可以针对性地对创面进行保护并促进创面愈合,目前已在创面修复领域得到广泛应用,是临床创面治疗的重要工具之一。本文分别就止血类、抗菌类、抗炎类、促血管化类及调控创面微环境类功能材料在创面修复中的应用做一总结。
【关键词】皮肤;伤口愈合;功能材料;创面微环境
基金项目: 国家自然科学基金重点国际合作项目(81920108022);国家自然科学基金重点项目(81630055)
Application of functional materials to promote cutaneous wound healing
Luo Gaoxing, Liu Menglong
State Key Laboratory of Trauma, Burns and Combined Injury, Institute of Burn Research, the First Affiliated Hospital of Army Medical University (the Third Military Medical University), Chongqing Key Laboratory for Disease Proteomics, Chongqing 400038, China Corresponding author: Luo Gaoxing, Email: 该Email地址已收到反垃圾邮件插件保护。要显示它您需要在浏览器中启用JavaScript。
[ Abstract ] Cutaneous wound is one of the common clinical diseases. Functional materials can provide targeted wound protection and promote wound healing through the structural adjustment and functional integration. Currently, functional materials have been widely used in the field of wound repair, becoming one of the important tools for clinical wound treatment. This paper summarizes the application of functional
materials of following categories including hemostasis,antibacterial, anti-inflammation, vascularization, and regulation of wound microenvironment in wound repair.
[ Key words ] Skin; W ound healing; Functional materials; W ound microenvironment
Fund program: Key International Cooperation Program of National Natural Science Foundation of China (81920108022); Key Program of N ational Natural Science Foundation of China (81630055).
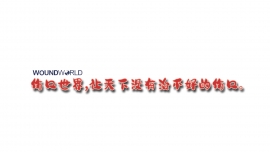
- 星期二, 13 9月 2022
利用表皮干细胞治疗兔角膜缘干细胞缺损
金 岩F! 刘 源 *! 张 超*! 董 蕊*! 雷 娟*
(第四军医大学组织工程研发中心, 西安 710032
摘要: 目 的 利 用 自 体 表 皮 干 细 胞 与 异 体 角 膜 基 质 细 胞 在 体 外 构 建 双 层 组 织 工 程 角 膜 ! 并 修 复 兔 角 膜 缘 干 细 胞 的 缺 损 " 方 法 建 立 兔 角 膜 缘 干 细 胞 缺 损 模 型 ! 以 去 细 胞 猪 角 膜 基 质 片 作 为 支 架 材 料 ! 以 自 体 表 皮 干 细 胞 与 异 体 角 膜 基 质 细 胞 作 为 种 子 细 胞 ! 在 体 外 构 建 组 织 工 程 角 膜 ! 并 用 来 修 复 兔 角 膜 缘 干 细 胞 缺 损 " 结 果 利 用 自 体 表皮 干 细 胞 与 异 体角 膜 基 质 细 胞 复合 异 种 去 角 膜 基 质 片 在 体 外 成 功 构 建 组 织 工 程 角 膜 # 构 建 的 组 织 工 程 角 膜 与 正 常 角膜 相 似 ! 具 有 上 皮层 和 基 质 层 # 用 组 织 工 程 角 膜 修 复 兔 角 膜 缘 干 细 胞 缺 损 T 个 月 后 ! 损 伤 角 膜 透 明 度 恢 复 良 好 ! 组 织 学 结 构基 本 恢 复 正 常 " 结论 成 功 构 建 了 兔 的双 层 组 织 工 程 眼角 膜 ! 并 修 复 了 兔角 膜 缘 干 细 胞 缺损 "
关键词" 表皮干细胞# 角膜# 组织工程# 角膜损伤
中图分类号:R 775.2
文献标识码:2
文章编号: 1000-503X(2005)06-0674-04
Treatment of Rabbit Corneal W ounds with Skin Epidermal Stem Cells
Jin Yan*, Liu Yuan*, Zhang Chao*, Dong Rui*, Lei Juan*
(Research Center for Tissue Engineering, the Fourth Military Medical University, Xi'an 710032, China)
Abstract: Objective To construct artificial rabbit corneas with autologous skin epidermal stem cells and allogenic stromal cells in vitro and promote healing of corneal wounds.
Methods: Skin epidermal stem cells were isolated from autologous skin samples. Keratocytes were isolated from newborn cornea biopsies. The cells were combined with acelular porcine corneal stroma scaffold to construct artificial corneas. Then the constructed artificial corneas were used to repair severe vision loss caused by complete loss of corneal epithelial stem cells.
Results: Cultured skin epidermal stem cells and keratocytes were in good growth conditions.Cultured artificial corneas consisted of multiplayer epithelial cells growing on stroma equivalent consisting of stromal matrix with incorporated keratocytes. The in vitro constructed artificial corneas were histologically similar to normal rabbit corneas. Three months after transplantation, the cornea wounds were healed and the rabbit cornea became transparent.
Conclusion: The artificial corneas were constructed successfully in vitro and can be used to repair severe vision loss caused by complete loss of corneal epithelial stem cells.
Key words: epidermal stem cells; cornea; tissue engineering; comeal wound Acta Acad Med Sin, 2005 ,27(6):674-677
Acta Acad Med Sin, 2005 ,27(6):674-677